Companion Guide to "Uncovering Diaphragm Cramp in SIDS and Other Sudden Unexpected deaths"
A plain-English summary of an emerging medical discovery in sudden infant death syndrome (SIDS)
Original article published in Diagnostics medical journal on October 18, 2024 (doi: 10.3390/diagnostics14202324) [1]. This SubStack incorporates newer information as our knowledge and understanding of diaphragm disease (hyperexcitability) evolves.
About our coauthored article that was peer reviewed and published in October 2024:
Compared to the other vital pump, the heart, the diaphragm is grossly understudied and under-appreciated. This opinion paper delves into the long-standing mystery of Sudden Infant Death Syndrome (SIDS) and its potential underlying mechanisms. The authors present a hypothesis centered around a newly recognized condition termed diaphragm cramp-contracture, which they suggest could be a significant factor in spontaneous respiratory arrests in children. Drawing from an extensive literature review and the unique case of a patient with a remarkable medical history, novel diaphragm cramp-contracture may elucidate some of the unexplained phenomena associated with SIDS.
For a quick summary of this lengthy post, jump ahead to Conclusions.
Introduction: A new SIDS hypothesis supported by highly compelling (if not convincing) evidence
The mystery behind SIDS (or cot death) appears to be unravelling. In a sentence: based on over two years of extensive literature reviews stemming from a patient with an extraordinary medical history, we have uncovered a novel cause of spontaneous respiratory arrests that is an elusive SIDS mechanism: diaphragm cramp-contracture (DCC). This plain English bullet-point list of essential findings raised in the above-titled opinion paper1 is slimmed down and geared for all readers, including lay persons, researchers and fellow physicians. Only the most pertinent research papers are cited. It also incorporates newer information, as our understanding of diaphragm disease is evolving. Important topics, hypotheses and SIDS prevention advice appear separately, like this:
It is important to point out that DCC has not yet been reproduced in lab or validated in vivo (in a living animal).
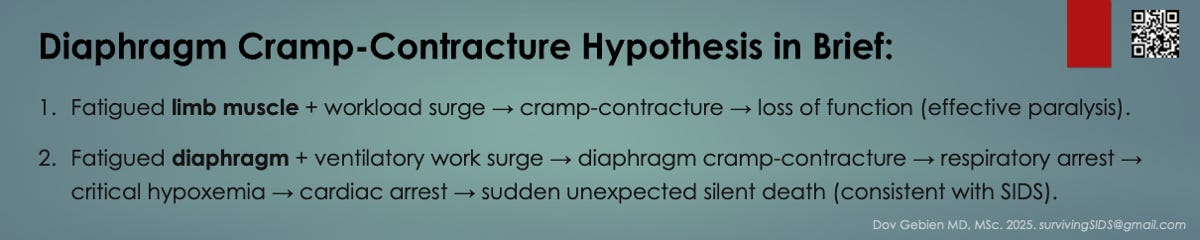
SIDS has probably plagued humankind for as long as we have existed: a 300,000-year-old mystery.
Approximately 8-10 children are found lifeless each day in Canada and the USA, combined, from SIDS and sudden unexplained deaths in childhood (SUDC), totaling 8.4 deaths per 10,000 live births from 2001-2019. The devastating losses, lifelong grief and unrelenting parental self-blame need no further discussion. Grieving families deserve a better explanation than what is currently available.
There are three leading SIDS hypotheses: (1) Triple-Risk (Filiano & Kinney 1994), (2) Infection-based (Paul Goldwater 2003)2 and (3) Critical Diaphragm Failure (Siren & Siren 2011)3. DCC incorporates all three, with particular emphasis on the latter. In fact, the Siren article helped set the groundwork for this paper.
Diaphragm Anatomy: This dome-shaped skeletal muscle, physically separating the chest (thoracic) and abdominal cavities, is the primary inspiratory muscle (see Video 1 and YouTube for anatomy animations). Inspiratory “neural” signals from the central nervous system (CNS), the brain, are carried by the left and right (bilateral) phrenic nerves, causing the diaphragm to contract bilaterally in inhalation (inspiration). As it descends towards the abdomen, the increasing chest volume (and lower pressure) creates an intrathoracic vacuum, expanding the lungs with air. When the diaphragm relaxes, exhalation (expiration) occurs passively, until a point in time called “end-expiration”, when the breathing cycle restarts.
Video 1: Anatomy of the respiratory diaphragm. When it normally contracts, it moves down, the belly expands and air enters the lungs. When it contracts in spasm, a variety of respiratory disorders can occur: forced expirations, hypoventilations (shallow breaths) and involuntary apneas (inspiratory arrest). See our YouTube diaphragm playlist for some high quality 3D anatomy animations.
Inexplicably, unlike the heart (the other vital pump), the diaphragm has been grossly understudied and unappreciated as causing serious symptoms in all ages, especially hypoxemic (low blood oxygen) episodes in infants, and sudden deaths in all ages from prolonged hypoxemia (causing cardiac arrest). Part of the problem is that it is an internal organ, and abnormal contractions are difficult to separate from normal ones. Also, diaphragm histology (tissue microscopy) is not routinely checked at autopsies. Or, if it is being done, abnormalities are written off as artifacts.
Furthermore, the diaphragm is often neglected when considering the causes of “brief resolved unexplained life-threatening events” in children of all ages, as well as certain (1) respiratory conditions (e.g. apneas and breath-holding spells, hypo- and hyperventilations, rib retractions, periodic and paradoxical breathing, oxygen desaturations and high CO2 levels, and even rapid breathing itself), (2) neurological symptoms (altered level of consciousness, fainting, visceral aura, and hypoxemia being both a cause and effect of some seizures) and (3) cardiovascular disorders (low and high blood pressures, low heart rates, new murmurs, pulmonary hypertension and sudden unexplained deaths).
Lastly, like the God-of-the-gaps principle, central nervous system pathology might have received too much credit explaining central and mixed sleep apneas, hypoxic ventilatory depression, autonomic seizures and cerebral edema as the final cause of death in ketoacidosis. Lopes et al. (1981) put it best, “The neural basis for apnea is so deeply entrenched that it is difficult to accept that some apnea may be due to respiratory muscle failure”4. In other words, diaphragm failure is causing serious symptoms and death (in vulnerable individuals) but is being missed.
Fortunately, the most vulnerable to spontaneous diaphragm failure, young infants, quickly outgrow the problem (typically starting at 6 weeks of life but may extend to 4 months). Respiratory muscle strengthening protects from failure.
New information presented here stems from a solitary patient, Patient 0, representing a sample size of only one (N=1). Medical breakthroughs do not normally happen this way, however, was the case with the antibacterial effect of fungal juice in 1928 that had inadvertently spilled on a bacterial culture plate.
Patient 0’s story is not fictitious. The ideas and evidence derived from his account are compelling and were abundantly supported by the medical literature.
Based on this now 53-year-old, healthy male physician patient who disclosed, what could be best described as spontaneous life-threatening cramp-like bearhug pain apneas awakening him from sleep sporadically from ages 7 to 17, using clinical (logical) reasoning we arrived at the diagnosis of simultaneous cramps of the diaphragm and intercostal muscles. Making a firm diagnosis based on medical history alone (without the need for confirmatory testing) was also unusual, let alone the delayed manner (45 years after the fact).
The unique combination of recurrent spontaneous bilateral (bearhug) rib pain with inspiratory arrest apnea and gasping during sleep (his mouth had opened involuntarily, unable to inspire) made it straightforward excluding other causes because they could not satisfy these criteria (see Table S1: Differential Diagnoses – for more). Furthermore, because he could not inspire despite trying, it appears there was also a total (complete) airway obstruction. Further details of his extraordinary story can be found in the Patient’s Perspective.
Diaphragm cramp (but not spasm) was medically unheard prior to our report. Perhaps because of mortality bias, whereby victims do not live to talk about it (let alone being recruited into research studies). Also, DCC is thought to affect infants and preverbal children most commonly; too young to remember. Lastly, rapid eye movement (REM) sleep is involved, the deepest sleep stage, making memory recall vague. For further speculation, see Table S2: Why Unknown to Medicine.
Despite the duress of severe unusual pain and imminent death, the 7-year-old had the presence of mind to experiment. He discovered DCC symptoms could be aborted by breathing out to breathe in: an expiration-inspiration, perhaps augmented by his three short burst, pursed-lip inhalations (see YouTube for his Rescue Breath Technique). With subsequent DCC events, he reused the same technique. However, he eventually recognized that painful twitches in his ribs (fasciculations) during sleep always preceded the full intensity bearhug cramp. If he quickly inspired before reaching end-expiration, the bearhug could be avoided. Lastly, given he learned to do this in his sleep, suggests he had endured numerous excitation events over his young life.
The fact that a 7-year-old child was able to keep calm, troubleshoot and then devise a counterintuitive impromptu solution while in pain, unable to breathe and alone in bed, is extraordinary. He likely would have perished if not for the rescue breaths.
Had he not autoresuscitated, critical hypoxemia would have ensued, leading to a rapid loss of consciousness (syncope, within 5-10 s), cardiac arrest (1-2 min later) and then death (in another 5 min), for a total duration of only 10 min, post-apnea. Brain injury would have started within 3 min of apnea.
Had Patient 0 perished, a diagnosis of “sudden unexplained death in childhood” (SUDC) would have applied given he was over one year of age. For those under 12 months, it would have been “sudden unexpected death in infants”, which includes sudden infant death syndrome (SIDS). SIDS is defined as the sudden death of an infant which remains unexplained after thorough investigation including a complete autopsy, death scene investigation and detailed clinical and pathological review [2]. SIDS is at least 40 times more common than SUDC and there is no evidence suggesting they have different causes. In fact, if they share a common mechanism, it would suggest a developmental vulnerability becomes outgrown with maturation, generally arising from a CNS, cardiac or respiratory cause. The latter includes the lungs and respiratory muscles but has received the least research attention. There are overlapping autopsy findings in SIDS and SUDC, and importantly, they involve the respiratory system (more below).
Low birth weight, preterm (premature) and younger full-term infants (under 4 months) are most vulnerable to respiratory muscle fatigue and failure5. Incompletely developed respiratory muscles and higher ventilatory workloads are primarily responsible.
Despite not being an infant at the time, many features of Patient 0’s apparent life-threatening events (ALTE) were shared with those known in SIDS. For instance, his death would have occurred silently and rapidly during sleep, and the preceding respiratory arrest had a sudden, unexpected and spontaneous onset with no preceding respiratory distress. Also, many risk factors overlapped with SIDS: male sex, night sweats (overheating), prone (face down) sleeping position (rebreathing), pulling loose blankets over shoulders or head (overheating and rebreathing), smoking household (nicotine exposure) and a colder geographic climate.
SIDS research has long suggested a respiratory cause. One involving airway obstruction (like Patient 0 had experienced):
In nine preterm infants aged 1-6 months who had basic home cardiorespiratory recordings of their final moments, seven had terminal apneas, gasping and evidence of airway obstruction just before death (Poets et al. 1999)6. Symptoms identical to Patient 0’s.
Respiratory observations in future SIDS victims compared to healthy babies revealed more sleep-related apneas, as well as apneas and intermittent airway obstructions while bottle feeding (not related to coughing or choking)7. In another study confirming the first, the obstructed breaths occurred mainly during REM sleep (78% of events), were accompanied by drops of heart rate and oxygen saturation levels down to 75% (hypoxemia) and associated with profuse night sweats while sleeping8. [Night sweating is a known SIDS risk factor. Cyanotic hypoxemic episodes and SIDS-like awake deaths have both been reported with bottle feedings.]
Autopsy evidence in SIDS, SUDC and seizure deaths: intrathoracic point-like (dot) hemorrhages (Tardieu petechiae), pulmonary congestion (heavy, fluid-filled lungs) and edema (frothy, blood-tinged airspace fluid) and gastric contents found in the mouth and airways. To produce these findings, a struggle to breathe against airway obstruction (agonal respirations) had likely created extremely negative intrathoracic air pressures, shunting blood into the lungs at elevated pressure (causing pulmonary hypertension and edema), while exposing the stomach to high intra-abdominal air pressures.
Despite intense effort and massive resources poured into SIDS research since the 1990s, the cause of this obstruction has never been found. Research shifted to the CNS but with disappointing results to date.
Diaphragm Contraction Abnormalities (Hyperexcitability Disorders)
The medical literature surprisingly revealed a host of disorders in all ages, many obscure, featuring involuntary diaphragm contractions. However, hiccups represented a more commonly experienced symptom (generated by brief diaphragm spasms). Both spontaneous and traumatic forms of excitation existed. Collectively, we categorized them all as, “diaphragm hyperexcitation disorders” (DHD), seen in the slide below.
It is important to note that mortality bias could have prevented us from appreciating the full extent of DHD presentations, particularly DCC, given it is thought to be immediately fatal. If one suddenly perished unwitnessed, the true cause would be missed and the diagnosis classified, again based on age, as SIDS, SUDC or SCD (or sudden unexpected death in epilepsy or traumatic cardiac arrest).
The causes of DHD are complex, complicated and beyond the scope of this paper. Associated medical conditions include chronic disorders of the neurological, neuromuscular and respiratory systems, as well as infections [e.g. viruses like COVID-19, influenza and respiratory syncytial virus (RSV)], electrolyte and acid-base disorders, the postpartum state, and not uncommonly, psychological distress. Many of these overlap with the causes of respiratory muscle weakness (see below).
Diaphragm Spasms (DS): Spontaneous brief (1-2 s) contractions in all ages, primarily preterm babies, that can run in succession up to 30-60 s at a time, causing worrisome oxygen desaturations. Symptoms include innocent hiccups and other gastrointestinal symptoms in some individuals, whereas persistent hiccups, forced expirations and apneas-hypopneas (shallow respirations) in others. Traumatic DS has been reported in abdominal winding injuries (celiac or solar plexus syndrome), where there is a blunt blow to the upper abdomen (epigastrium) or chest, commonly seen in martial arts for example9. Like spontaneous DS, a forced expiration may occur, followed by an involuntary apnea which inevitably triggers panic because the victim cannot breathe in for a few seconds. Sometimes, the victim might even collapse (syncope), probably from severe hypoxemia. See Traumatic DCC just below for sustained DS, which is thought to have the worst prognosis. [Skip to Winding Injuries]
Diaphragm Flutter (DF, van Leeuwenhoek’s Disease): Spontaneous sudden-onset rhythmic diaphragm contractions in all ages lasting minutes to hours that can be episodic. Both low and high frequency forms exist: 20-480/min versus 500-900/min, the former similar to cardiac atrial flutter whereas the latter to ventricular fibrillation. Antonie van Leeuwenhoek was the first to describe it in 1723 when he wrote, “I thought I was at death’s door. I was seized by a violent movement around that large and vital organ we call the diaphragm, so much indeed that those standing around were not a little alarmed”10. He died suddenly later that same year, reportedly from DF. Although symptoms in adults may include epigastric/chest pain and bulging epigastric pulsations, respiratory distress is most common, sometimes with pale skin (pallor) or blue discolouration (cyanosis) requiring assisted breathing (CPAP or mechanical ventilation). DF in infants can be asymptomatic11 and so might be more common than currently thought. Specialized studies are needed to detect it: respiratory inductive plethysmography (RIP), electromyography (EMG) or fluoroscopy. In others, life-threatening respiratory failure occurred [Panigrahy 2012, Riordan 1990].
Respiratory Flutter (RF): Identical to DF, also in all ages, but other respiratory muscles contract simultaneously with the diaphragm, including the intercostal (between-rib), neck (scalene) and abdominal wall (rectus abdominae) muscles. Three newborns with RF had respiratory distress requiring short-term assisted ventilation. The clustering of cases over a short period of time prompted the authors to declare that “RF was an under-recognized cause of respiratory failure in neonates”12.
Diaphragm and Respiratory Myoclonus: Like DS and DF/RF but with brief bursts of arrhythmic diaphragm ± accessory respiratory muscle contractions, sometimes with abdominal muscle pulsations. Epigastric/chest pain and disabling shortness of breath have been reported. More than one paper on the subject mentioned it may belong to a spectrum of abnormal diaphragm contractions.
Belly Dancer’s Dyskinesia: Slow contorting, writhing contractions of the rectus abdominae muscles, causing the umbilicus to move slowly in all directions. It remains uncertain, however, if diaphragm contractions actually accompany these.
Sustained Contractions Causing Acute Diaphragm Paralysis (ADP) and Putative Diaphragm Cramp-Contracture (DCC): An effective form of ADP results from sustained diaphragm contractions, whether by spontaneous cramp-contracture or exogenous factors. This causes a prolonged apnea respiratory arrest and represents a peripheral form of failure (compared to central and obstructive apneas). Severe hypoxemia rapidly follows, causing secondary cardiac arrest and death if not aborted or resuscitated*. ADP may occur in spinal cord injuries of the neck, electrocutions and seizures (the latter was experimentally induced in mice) as well as with neuromuscular blockers like nicotine, succinylcholine and neurotoxins (e.g. curare, tetanus). In addition, DCC-ADP may occur in some high energy blunt abdominal winding injuries, including motor vehicle collisions, falls from heights and high-impact bodily collisions.
*A near-fatal cardiac arrest occurred in the Damar Hamlin NFL injury (2023), in which Mr. Hamlin collapsed after colliding with another player. On one particular video angle, he appeared not to have seen the imminent collision and did not brace himself in time. A shockwave could be seen on slow-mo replay traversing his body before he fell. He then stood up trying to remove his helmet but fell backwards and apparently lost consciousness. We proposed his diaphragm may have absorbed kinetic energy from the force of the collision, triggering DCC respiratory arrest. Rapidly ensuing hypoxemia could have caused the syncope, followed by secondary cardiac arrest (not primary, as commonly held). Fortunately, CPR was immediately started, and paramedics apparently brought his pulses back. Although commotio cordis was thought causal (primary cardiac arrest), that involves a projectile hitting the chest and occurring directly over the heart, which did not happen.
Nicotine is a highly potent neuromuscular blocking agent that induces rapid respiratory arrest at all ages by ADP (not CNS-induced). Deaths may occur in 5-30 min, even from minute ingestions (snuffbox scrapings in one case, a 6-year-old). This is important because of the ubiquity of “vape” liquids and that tobacco smoke exposure is a SIDS risk factor. Younger children, especially infants, are most vulnerable to absorbed nicotine.
SIDS Hypothesis: Nicotine absorption from household tobacco smoke in sleeping infants with diaphragm fatigue causes peripheral respiratory arrest by reducing the DCC threshold.
Nicotine is exceptionally dangerous in small children, even minute amounts. It is important to keep vapor liquids safely stored and do not smoke anywhere near an infant or in the household. But if so for some reason, then do not let the child sleep upstairs (smoke rises).
To prevent rebreathing of exhaled gases, use a bedroom fan and slightly ajar window to gently stir the air. No bed companions, loose blankets, bed toys or sleeping face down (prone).
Do not allow the child to sleep on your chest or on the sofa, even for a short nap (SIDS cases have occurred this way).
Breathing Mechanics, Respiratory Weakness and Failure
The diaphragm is the primary inspiratory muscle. It is composed of tendon and skeletal muscle.
Inspiratory muscles also include the respiratory accessory muscles (RAM), which reduce diaphragm workload [4]. With inspiration in adults they expand the ribcage, whereas in infants, protect the ribcage from deformity (because of its pliable, cartilaginous structure). Plasticity is a major fatiguing factor in younger infants but fortunately, improves with maturation, by bone ossification (hardening).
The external intercostal muscles (ICM) are the primary inspiratory RAM. Others include the scalenes and sternocleidomastoids of the neck, and pectoralis muscles of the chest, but not the abdominal muscles (which assist expiration only).
Normally, the relative diaphragm-to-RAM contributions to the work of breathing (WOB) is about 80:20. However, RAM workload increases under higher demands such as exercise, obstructive sleep apnea and respiratory infections [from airway congestion, wheezing, lung consolidation, or small airway collapse (atelectasis) for example].
Respiratory infections are categorized as upper airway (URI), such as the common cold, pharyngitis, laryngitis and bronchitis; or lower (bronchiolitis and pneumonia). In addition to increased WOB from added airway resistance, the latter two also impair gas exchange in the lungs, further increasing respiratory rate, metabolic demand and consequent diaphragmatic WOB.
Ventilatory workload becomes diverted to RAM as diaphragm fatigue sets in (and vice versa). This is known as respiratory load sharing (or load dependence) [4]. ICM contractions become visible as rib retractions (sucking in of the intercostal soft tissues). As they tire (or fail), work is diverted back to the diaphragm (and is hereby proposed to be the mechanism of periodic breathing: alternating cycles of respiratory muscle group fatigue and failure, followed by load-dependent compensation; see below). Also, paradoxical breathing can develop from severe diaphragm fatigue (and failure). With inspiration, the chest wall contracts instead of expanding whereas the abdomen moves up and in instead of bulging down and out. This is worrisome because total respiratory failure can suddenly ensue (sustained apnea from simultaneous failure of both muscle groups).
Paradoxical breathing is a medical emergency. Also, although rib retractions on their own may not be, if they occur with rapid breathing, sweating, fevers, hiccups or any form of distress, have your child seen by a doctor ASAP.
The causes (etiologies) of inspiratory muscle weakness in all ages determined upon literature review are summarized in Table 1. They may occur suddenly (acutely) or gradually over a long period of time (chronic). Some are irreversible but sometimes may improve over time (e.g. phrenic nerve injuries). Importantly, nicotine exposure and electrolyte disorders are known SIDS risk factors.
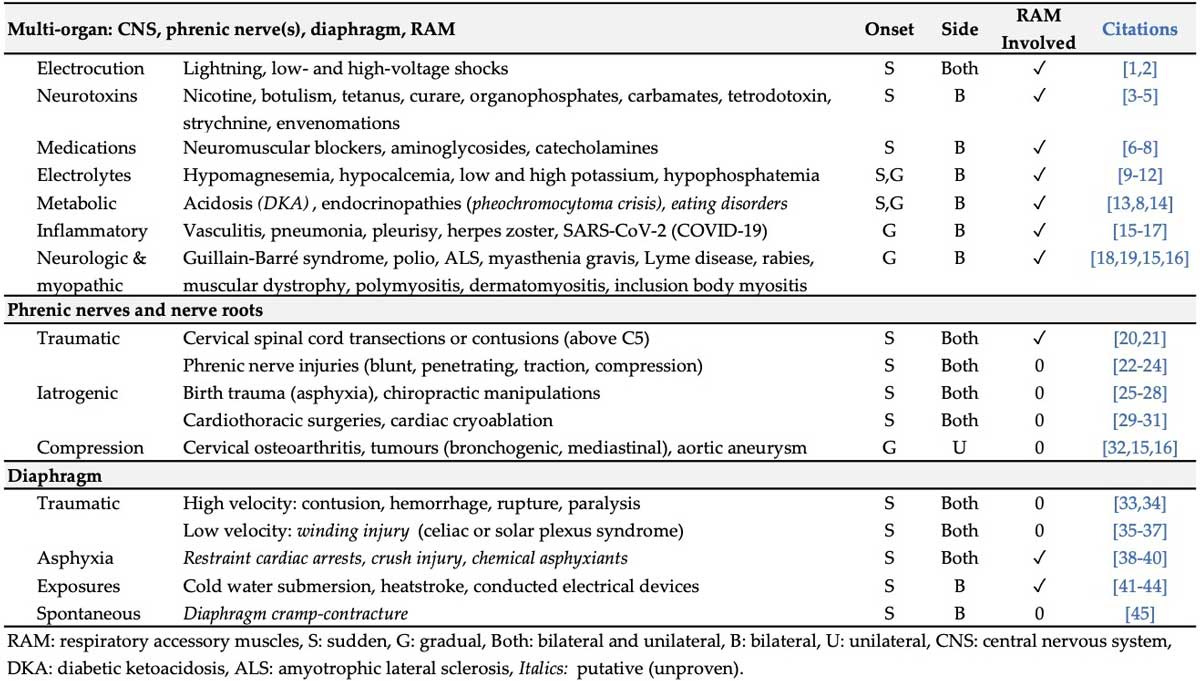
Examples of medical conditions causing chronic inspiratory muscle (diaphragm) weakness included progressive neurodegenerative (neurological) disorders or myopathic diseases like muscular dystrophy, whereby skeletal muscles of the limbs, trunk and diaphragm become weaker over time.
In advanced stages, life-threatening respiratory muscle weakness (and arrest) can suddenly develop. This may occur from an added ventilatory workload on the diaphragm (or heart), even by a typically nonlethal URI. Increased airway resistance and an increased respiratory rate (tachypnea) in response to infection, pleural effusion or congestive heart failure could tip the balance.
Acute inspiratory muscle weakness may also cause sudden deaths. Examples included injuries to the spinal cord or bilateral phrenic nerves, both which can paralyze the diaphragm (i.e. ADP). Similarly, diaphragm injury (myopathy) from COVID-19 virus was confirmed at autopsy in adult ICU patients who had died by rapid respiratory failure13. Progressive muscle damage of diaphragm and RAM had occurred, likely leading to weakness and failure. Other examples were exposures to neurotoxins (already mentioned), venoms (snakes, spiders, jellyfish) and extreme heat, such as vehicular heatstroke and malignant hyperthermia (an adverse effect of anesthesia medications like succinylcholine). Death in children exposed to extremely high temperatures might have been by diaphragm failure [Razuin 2020]. Autopsy findings overlapped with SIDS [Krous 2001] and malignant hyperthermia myopathy [Christiansen 2004].
From this knowledge, we determined that rapid onset respiratory muscle weakness from all causes was an underrecognized cause of serious morbidity (respiratory distress and apneas) as well as mortality in all ages (sudden deaths by ADP respiratory arrest).
One particularly heartbreaking case was a 13-year-old girl who tripped and fell in gym class while trying to jump a hurdle, striking the base of her head on the crossbar14. She died immediately. At autopsy was a large neck contusion (collection of blood and swelling under the skin). There was no visible spinal cord injury explaining the sudden death, so the authors hypothesized she had died from a severe concussion. However, given our fresh perspective, we considered that bilateral phrenic nerve root compression, stunning (neuropraxia) or stretching (traction) might have caused ADP respiratory arrest.
Hypothesis: Acute diaphragm paralysis can be immediately fatal when it is bilateral, neurologically complete (inactivates the diaphragm completely) and occurs in those with weak, paralyzed or cramped RAM (failure to take over the work of breathing).
Putative DCC in very young infants satisfies this criteria because they have underdeveloped, untrained RAM.
RAM recruitment and training otherwise occur with maturation, especially if: (a) diaphragm weakness starts gradually, (b) it is unilateral or (c) is partial (i.e. incomplete diaphragm paralysis).
In the 13-year-old child above, we speculated her RAM became paralyzed along with the diaphragm. In addition to the bilateral phrenic nerve disruptions, sudden neck flexion-extension may have disrupted the nerves supplying her bilateral ICMs (the intercostal nerves).
Also, for ADP to be fatal, sufficient time needs to elapse for the hypoxemia to induce cardiac arrest and death (1-2 min and 10 min, respectively). So, the ten-minute window offers an opportunity for resuscitation.
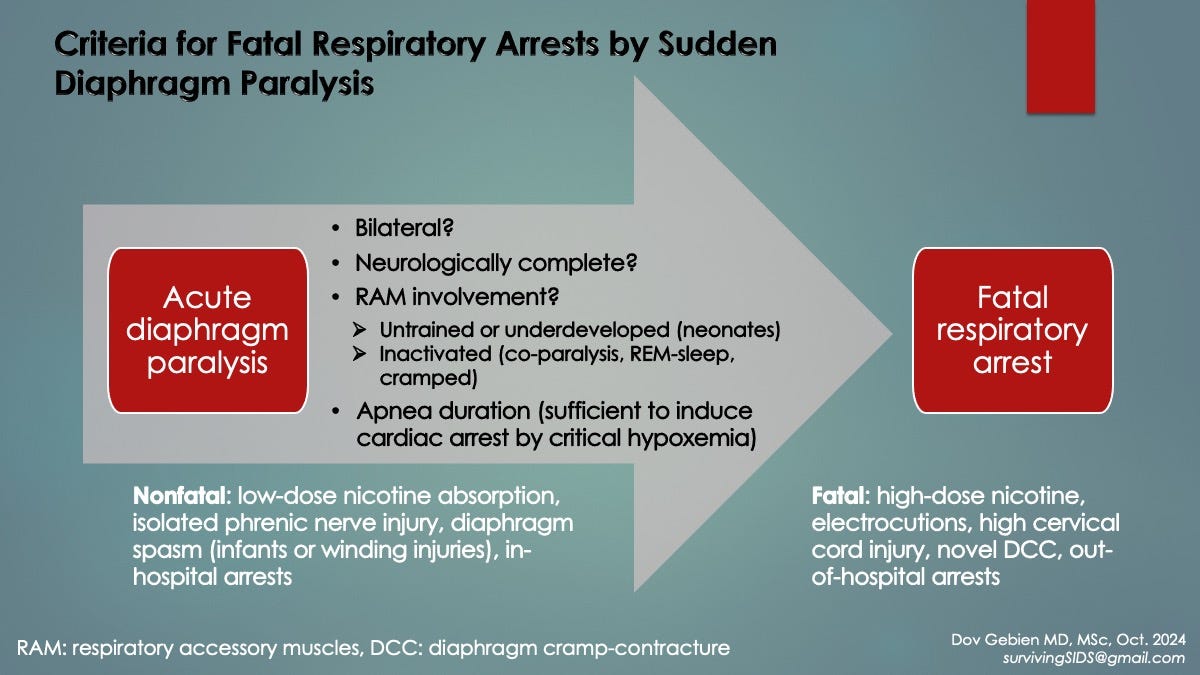
Supporting evidence: Experimental paralysis of the diaphragm (by severing the bilateral phrenic nerves) caused rapid death in newborn (neonatal) test animals, whereas older infants survived. Hypothesis: Their more mature RAM were able to resume work of breathing independent of the diaphragm, whereas neonatal RAM did not.
This demonstrates how, as compared to older infants, the very young (and premature) are highly vulnerable to respiratory failure deaths: because of weak, incompletely developed, untrained respiratory muscles. It also supports our claim that RAM in young infants cannot independently ventilate the lungs when breathing against an inactivated diaphragm.
Diaphragm Fatigue, Hypertonicity and Excitation
Unlike muscle weakness, fatigue improves with rest. Contrarily, if muscle rest becomes incomplete, fatigue worsens.
Limb and trunk muscles can rest when inactive, however, this is not possible with the diaphragm because of the continuous nature of the respiratory cycle (alternating diaphragm contraction and relaxation). Also, rest can only occur during the expiration phase, again reducing available time for muscle recovery. This becomes even more problematic under increased respiratory rates, say during exercise or illness, when there is less expiration time. As a consequence of incomplete diaphragm relaxation, diaphragm fatigue becomes a serious issue and manifests as increased muscle tone (hypertonicity) and reduced contractility. In fact, the hypertonicity could serve as a surrogate marker of diaphragm fatigue (which has been measured in preterm babies using diaphragm EMG [Plante 2023]).
Another issue contributing to incomplete rest/fatigue is that skeletal muscle relaxation times become prolonged under certain conditions: (a) higher workloads (lusitropy), (b) hypoxemia, (c) hypercapnia, (d) acidosis and (e) bacterial endotoxins. For the diaphragm, this appears to be an adaptive response to maintain respiratory system homeostasis (balance), however, has its limits. When overcome in skeletal muscles or the heart, hypertonicity and pump failure respectively ensue.
Other outcomes of severe skeletal muscle fatigue include excitation-contraction uncoupling, loss of membrane integrity (muscle cell damage) and electrolyte imbalances [e.g. potassium (K+), calcium (Ca+2), magnesium (Mg+2), phosphate]. This is important because these may serve as surrogate biomarkers of critical fatigue measurable in blood samples, particularly creatine kinase (CK).
When blood supply of oxygen or other metabolic substrates (like glucose and electrolytes) to the diaphragm become outpaced by demand, metabolic mismatch occurs. Accumulation of metabolic byproducts also occurs [including acidic hydrogen ions (causing local acidosis) and phosphates]. Contractile dysfunction ensues, forcing the organ to work harder to maintain homeostasis, thus contributing to diaphragm fatigue.
Under increased workloads, fatigued limb and trunk muscles become prone to neuromuscular excitation (as cramps). This is experienced by us all, especially when unfit, overheated or dehydrated (with acidosis). Excitation is triggered upon initial shortening (activation) of the contracting muscle.
Examples of neuromuscular excitation include transient twitches (fasciculations and spasms), myoclonic bursts and sustained cramp-contractures.
The diaphragm too is vulnerable to excitation; however, less is known about it. Unlike a limb cramp, the diaphragm is internally located, so spasms and cramps cannot be aborted by stretching [yet Patient 0’s rescue breath technique somehow did overcome it].
Young infants (under 4 months’ age), compared to older ones, are especially vulnerable to diaphragm fatigue because of numerous innate factors that diminish with maturation: a plastic-like, compliant ribcage (increased WOB), weaker, less developed respiratory muscles (strained under higher respiratory rates/demand), less fatigue-resistant diaphragm muscle fibers, higher metabolic rate and resting respiratory rates, and smaller lung airspaces (prone to occlusion by lung infection, atelectasis and secretions, all causing an added WOB ).
Numerous diaphragm fatiguing factors in young infants are thought to act (and interact) synergistically, raising the potential for diaphragm excitation. We used the term, critical diaphragm fatigue, to describe when the threshold for excitation is exceeded. All that is needed to trigger it is a workload surge, a sudden reduction in muscle contractility, if relaxation time becomes abruptly delayed or expiration phase (rest time) is shortened (i.e. from sudden tachypnea).
Table 2 (below) lists the wide variety of conditions in infants contributing to respiratory muscle fatigue and added WOB. As opposed to muscle weakness factors in Table 1, these are generally reversible or temporary. Many overlap with known SIDS risk factors. This supported our (and others’) SIDS hypothesis that critical diaphragm fatigue and workload surges predisposed some infants to ventilatory failure by DCC.
[These are identical tables (above and below)]
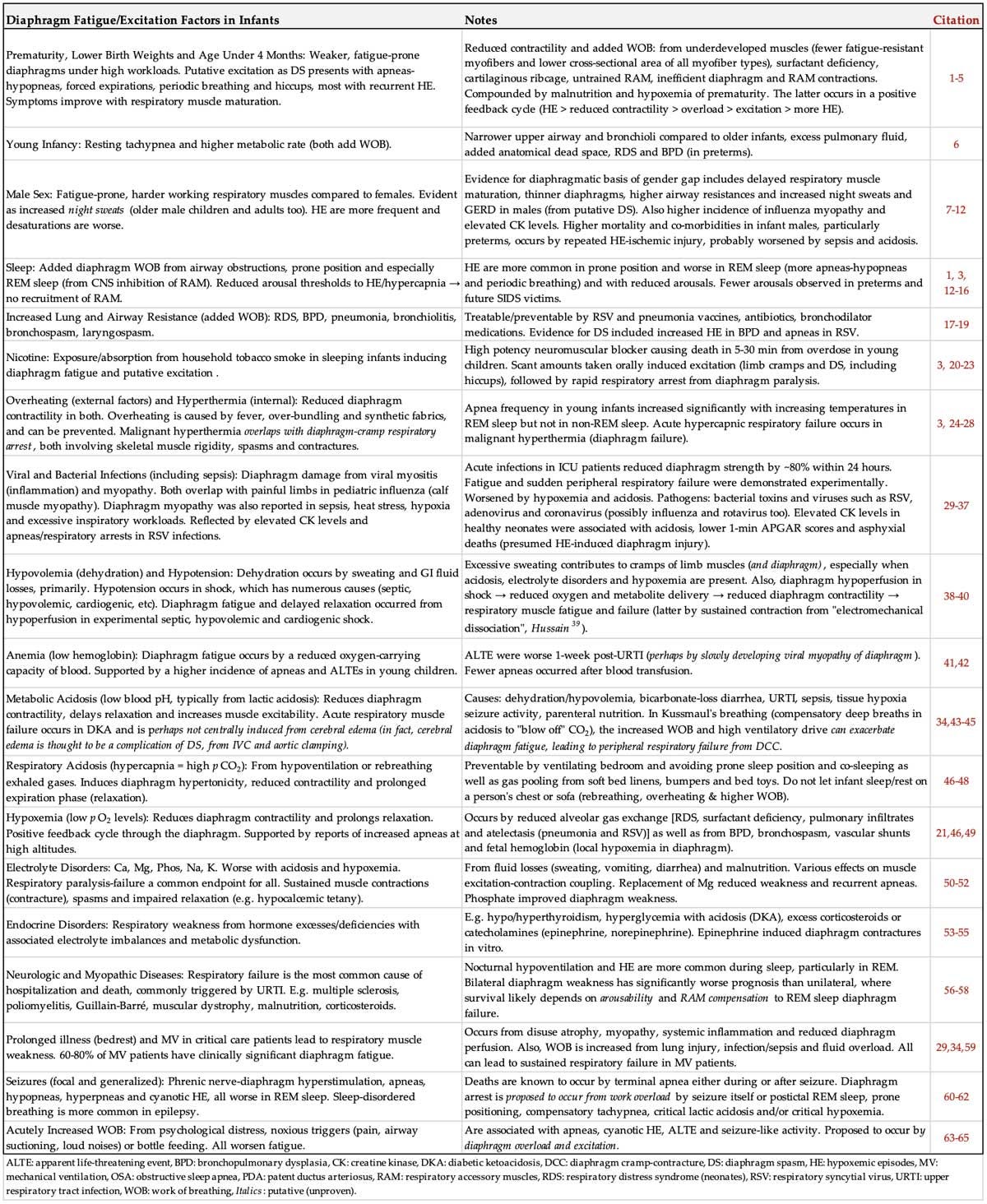
Critical acidosis (and extreme hyperkalemia) were reported at SIDS autopsies in 2006 but never corroborated. These commonly accompany severe bacterial infections and sepsis and have detrimental effects on skeletal muscles, perhaps lowering excitation threshold like in ventricular fibrillation15.
Another important fatiguing element causing contractile dysfunction was viral-induced diaphragm inflammation (myositis) and injury (myopathy) [see Diaphragm Injury at Autopsy, below]. Cases were reported in children with RSV, rhinovirus, adenovirus and Coxsackie virus, and in adults with COVID-19. RSV tends to have more severe symptoms than other respiratory viruses and is known to present with apneas and sudden unexpected deaths in young infants16. Other respiratory viruses were suspected by us, particularly influenza, because of its association with pediatric calf muscle myopathy. Gastrointestinal viruses might also infect the diaphragm, such as rotavirus and norovirus. Apneic episodes and sudden unexpected deaths have been reported in rotavirus [Riedel 1996]. However, to our knowledge, viral pathology reports specific to the diaphragm were not available to confirm this.
In REM sleep, to avoid physically acting out dreams, the CNS has evolved to inactivate limb and trunk skeletal muscles by tonic inhibition (sleep atonia). A dreaming dog in REM sleep serves example, as exhibited by muffled barking and restricted running movements. Although the diaphragm is spared from atonia, RAM and upper airway “dilator muscles” are not (the latter traditionally held to cause OSA). In both cases, a sudden ventilatory workload is placed on the diaphragm. This could be problematic when it is already fatigued.
Diaphragm workload surges are known to arise from REM sleep onset and sudden-onset tachypnea. We added a position change to prone, worsening infection (diaphragm myositis/myopathy) and diaphragm-stimulating seizures. CNS tachypnea occurs with respiratory and gastrointestinal infections, noxious stimuli like pain and loud noises, as well as intense emotions like anger or anxiety. Severe cyanotic/hypoxemic episodes in infants were more common when household tension was high [17]. Infection, particularly respiratory, increased the frequency and severity of events, probably because added fatigue and workload triggered diaphragm excitation.
Avoid startling a baby with loud noises, arguments or anything else that might increase household tension. It can trigger a breathing emergency.
In a supine-sleeping infant (face up) with critical diaphragm fatigue, diaphragm excitation may be triggered by REM sleep workload surges (i.e. inactivation of RAM and airway dilator skeletal muscles). In other words, REM sleep could represent a SIDS catalyst. This is consistent with the nocturnal, sudden and unpredictable nature of SIDS as well as with Patient 0’s sudden respiratory arrests.
It is clear then in infants, a complex interplay of respiratory weakness and dynamic fatiguing factors superimposed on heightened vulnerability to fatigue contribute to potential diaphragm overload by a workload surge that catalyzes neuromuscular excitation as brief DS apneas and sustained contraction DCC respiratory arrests that could cause SIDS-like deaths.
Hypotheses (summary):
In young infants, respiratory muscle weakness from incomplete development + cumulative effect of fatiguing factors + limited ability of diaphragm to rest → increased risk for critical fatigue. This is when the threshold for excitation is exceeded and all that is needed to trigger it is an added workload.
1. In all ages, critical diaphragm fatigue + ventilatory workload surge → excitation → diaphragm spasms (DS, including hiccups), myoclonus (DM), flutter (DF) or cramp (DCC). It is unknown why one form occurs over another; however, DS appears to be the most common. Varying arousal thresholds from sleep hypercapnia in infants may play a role (i.e. hypercontraction stops with waking up, followed by reactivation of RAM, deeper breaths and increasing oxygen levels).
2. Diaphragm fatigue → incomplete relaxation after each contraction → increased muscle tone (hypertonicity) → diaphragm excitation
3. Incomplete relaxation → lung air trapping (breath stacking) → hyperinflation → diaphragm thinning → mechanical disadvantage → diaphragm pump insufficiency → lung alveolar hypoventilation → hypoxemia and hypercapnia → more fatigue (positive feedback cycle).
4. Reduced diaphragm blood supply (perfusion) in tachypnea → diaphragm fatigue → hypoxemia and hypercapnia → worsening tachypnea + reduced perfusion? (positive feedback cycle).
Respiratory Instability (RI) in Preterm (Premature) Infants
Given SIDS affects young infants most commonly (age peak at 2-4 months old), we explored the medical literature on early infancy. Unsurprisingly, respiratory pathology is the primary issue and worse with younger age, lower birthweight and increased prematurity. Many preterm babies require extended periods of MV in the neonatal ICU as a result. Also, males are affected more often and more severely than females (see Male Preponderance below). Fortunately, most appear to outgrow it by the fourth week of life. This too supported the notion that incomplete respiratory development is associated with SIDS. Our hypothesis diverged from mainstream ideas concerning CNS immaturity and central apneas. Incomplete respiratory muscle development with consequent vulnerability to fatigue, overload and sudden failure represented peripheral apneas, not central.
Respiratory instability (RI), a general term describing a variety of breathing abnormalities observed in infants in the ICU, is associated with repeated hypoxemic episodes (HE)171819. These are generally transient (brief), sometimes associated with silent squirming and include: forced high-volume expirations (hyperpneas), apneas (most under 10 s but worrisome at 20 s), hypopneas (shallow breaths from airway obstruction), pallor/cyanosis, bradypneas (reduced respiratory rates), bradycardias (low heart rates, typically following apneas-hypopneas), periodic breathing (alternating cycles of tachypnea and brief apneas) and/or increased arousals (fragmented sleep). Hiccups, which are more common in the youngest infants, could also be included here especially given spells occur often, and many are followed by apneas-hypopneas20.
Although the cause of RI has never been elucidated (only speculated to be CNS immaturity, intermittent airway obstructions of uncertain origin or abdominal muscle contractions), the pathologic agent, nonetheless, is intermittent HE. Although brief HE appear to be tolerated, they can be dangerous, both immediately (when repeated or prolonged), or collectively, accumulating over the course of several weeks to months [if not over a lifetime]. In other words, there are serious short- and long-term complications of hypoxemic episodes in respiratory instability.
Although most HE occur without overt signs of infant distress [because of short duration], others may present with a variety of gastrointestinal and neurologic symptoms (in addition to respiratory): gastroesophageal [acid] reflux disease (GERD) and feeding difficulties, loss of muscle tone (hypotonia or floppiness), back arching and other unusual posturing, seizures and/or loss of consciousness. It is important to realize that unwitnessed crib deaths may also be a presentation (i.e. SIDS). While it is known that hypoxia causes seizures, it appears the opposite holds true, whereby seizure activity can trigger HE (via the diaphragm; see SUDEP below for more).
Frequent hiccups in an infant could indicate critical diaphragm fatigue. See a doctor immediately if there is fever, respiratory infection, diarrhea or any breathing problems (i.e. rapid rate, pale or bluish skin, breathing pauses or rib retractions).
Literature review indicated HE may strike when a baby was awake or asleep and are worse in REM sleep (more frequent and prolonged). They were also more common when bottle feeding and if respiratory infection was present [7,8,17].
Using surface EMG of diaphragm and ICM, Lopes et al. determined that diaphragm fatigue existed in 12 of 15 otherwise healthy preterm infants at 2 weeks’ age [4]. Also, apneas involved simultaneous failure of both muscle groups (preceded by escalating fatigue) and were triggered by REM sleep (mechanism unknown). Furthermore, apneas persisted when ICM did not resume the work of breathing, suggesting a peripheral role for impaired arousal (i.e. not waking from REM sleep to reactivate ICM).
Defined as oxygen saturations under 88% for over 10 s (normal is above 96%), HE can range from tens of seconds to 2 min and may occur hundreds of times daily (a cumulative danger). Saturations can even drop below 70% in some cases and for several minutes at that; a respiratory emergency given hypoxic brain injury begins at 3 min.
Short term complications (morbidity) of recurrent HE in early infancy include cerebral hypoxia (causing seizures, hypotonia, brain injury and/or coma), brain hemorrhages (intraventricular), bronchopulmonary dysplasia (BPD), acute pulmonary hypertension, poor feeding/weight gain and increased risk of necrotizing enterocolitis (damaged intestines) and serious infections like pneumonia, meningitis and bacterial sepsis. Sudden respiratory arrests are also a concern in preterm infants, especially when co-morbid disease is present (i.e. cardiac, respiratory, neurologic, myopathic or metabolic conditions).
Long term morbidities of HE include visual and hearing deficits, growth and neuro-developmental delays and cerebral palsy (in birth asphyxia21,22) as well as chronic pulmonary hypertension, structural heart diseases (cardiomyopathies) and sudden respiratory arrests in the first 18 months of life from sudden unexplained deaths (consistent with SIDS and SUDC) [17,23]. Preliminary evidence in rat pups exposed to 90 min of postnatal hypoxemia suggested a connection to attention-deficit hyperactivity disorder (ADHD)24.
Hypothesis: Critical diaphragm fatigue with hyperexcitability is responsible for RI in infants and the HE and complications that follow them. REM sleep inhibition of accessory muscles is the primary trigger during sleep. Increased diaphragm fatigue and workload by respiratory infections may explain why HEs are more frequent when infection is present.
This hypothesis was best supported by infant studies employing gastric pressure sensors and abdominal surface EMG [using sticky leads like a heart ECG but measuring underlying muscle activity instead]. As can be seen in Fig. 1 below, preceding each HE (blue ellipse) was a burst of muscle contractions associated with forced expirations and transient apneas (red boxes). Instead of just the abdominal muscles, simultaneous diaphragmatic ± abdominal contractions could have been responsible (like respiratory flutter but as short twitches and spasms instead). If true, then this is one of the most important pieces of evidence connecting diaphragm excitation to RI and HE.
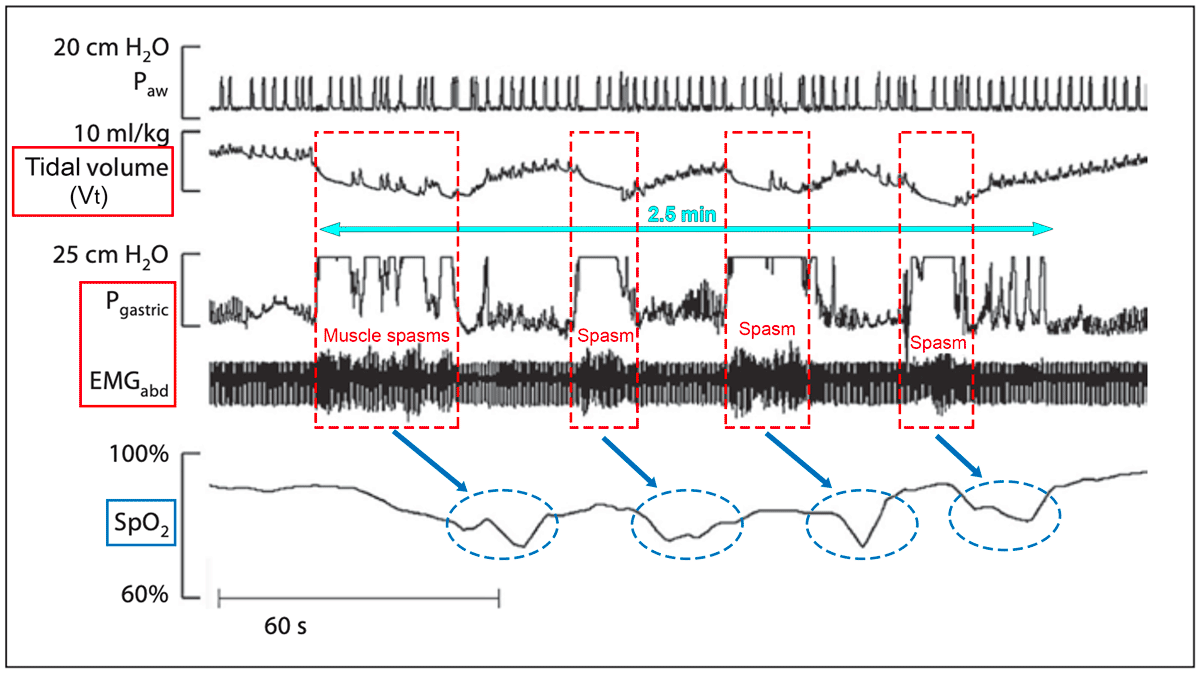
Periodic breathing too could be explained by repetitive cycles of diaphragm fatigue (with ensuing overload and DS) alternating with RAM fatigue (overload and spasm too), whereby workload is diverted from one group to the other via respiratory load sharing. In the image above, each brief apnea (Vt flatline) is associated with a DS spike and followed immediately by rapid breaths. The latter could reflect compensation to the hypoxemia (and hypercapnia).
Obstructive Sleep Apnea (OSA) and Diaphragm Excitation
OSA in older children (and adults) is a highly prevalent, underdiagnosed condition. It is often silent, so is missed by parents. Older individuals may not even know they have it, which presents a problem because of serious medical complications.
If you suspect breathing pauses or obstructed breaths in your sleeping child, have them seen by a pediatric sleep specialist for testing. A pediatric CPAP mask might be indicated.
Like RI in infants, OSA also involves repeated apneas and hypopneas. These too are more common during REM sleep, associated with night sweats and worse in males (overlapping SIDS risk factors). Night sweats might reflect higher heat output of harder working respiratory muscles. Frequent arousals also occur in OSA, waking up gasping or choking for several seconds.
Like RI, OSA too carries significant short and long-term morbidities and higher overall mortality. In adults these include systemic and pulmonary blood pressure surges (leading to a diagnosis of chronic hypertension), cardiomyopathies (abnormal heart chamber dilatations or wall thickenings) with resultant congestive heart failure (pump inefficiency causing pulmonary edema), heart valve disorders, coronary artery disease (angina and heart attacks), cardiac arrhythmias, stroke (acutely reduced cerebral blood flow), venous thromboembolism (blood clots, typically in the legs) and an increased risk for sudden cardiac deaths (SCD). In one study, SCD occurred most often during sleep hours (12-6 am) in those with OSA compared to SCD in control subjects, where the risk of SCD correlated to the severity of OSA (more apnea-hypopnea events).
OSA has historically been explained by reversible collapse of the upper airway dilating muscles during sleep. Instead, we suggest this is not the primary cause, but rather contributes to fatigue because of added airway resistance. Increased workload then triggers diaphragm spasms, causing the sleep apneas and hypopneas.
Like RI in infants, we propose OSA in older children and adults is caused by DS. That OSA and RI are one of the same.
The novel airway obstruction of DS in OSA develops when RAM contractions to breathe are resisted by the hypercontracted, incapacitated diaphragm. This produces the low volume hypopneas. Again, it can be triggered by added workload in REM sleep or from reversible soft tissue upper airway obstructions.
Evidence linking diaphragm pathology to respiratory problems in infants (with RI) and older children and adults (with OSA) was given by four important studies:
1. In 13 preterm infants with apneas, expiratory time and total pulmonary resistances increased in the breaths immediately preceding apneas that could not be fully accounted for by supraglottic resistance (i.e. constrictions of the upper airway dilator muscles or other soft tissues)25. Along with Southall’s findings of continued HE in infants despite tracheostomies or endotracheal tubes in place, upper airway obstructions were not the cause of the increased-resistance apneas. Something else had caused it (laryngospasm and bronchospasm were mentioned but not proven).
2. In 21 adults with OSA, activation of the respiratory muscles (airway dilators, ICM and diaphragm) was compared with those of healthy control subjects using EMG. All muscles were more active in those with OSA, both awake and asleep, reflecting a chronically increased diaphragm workload26.
3. In another adult OSA study by the same group, one polysomnogram showed a 30-s-apnea (shaded red box in Fig. 2 below) associated with sudden cessation of chest and abdomen ventilatory movements consistent with a central apnea (i.e. lack of “neurally stimulated breathing”)27. However, because diaphragm electrical activity did not cease (active in ‘EMG1-5’), the diagnosis was changed to obstructive apnea. The problem with that however, was obstructive apneas normally involve continued respiratory efforts, which were not present, so something else had to be responsible. The clue was in the D-EMG waveforms, reduced in intensity during the apnea. We believed peripheral malfunction (of the diaphragm) had taken place, whereby mechanical failure had occurred: despite phrenic nerve stimulation, the inspiratory muscles had failed, causing the HE.
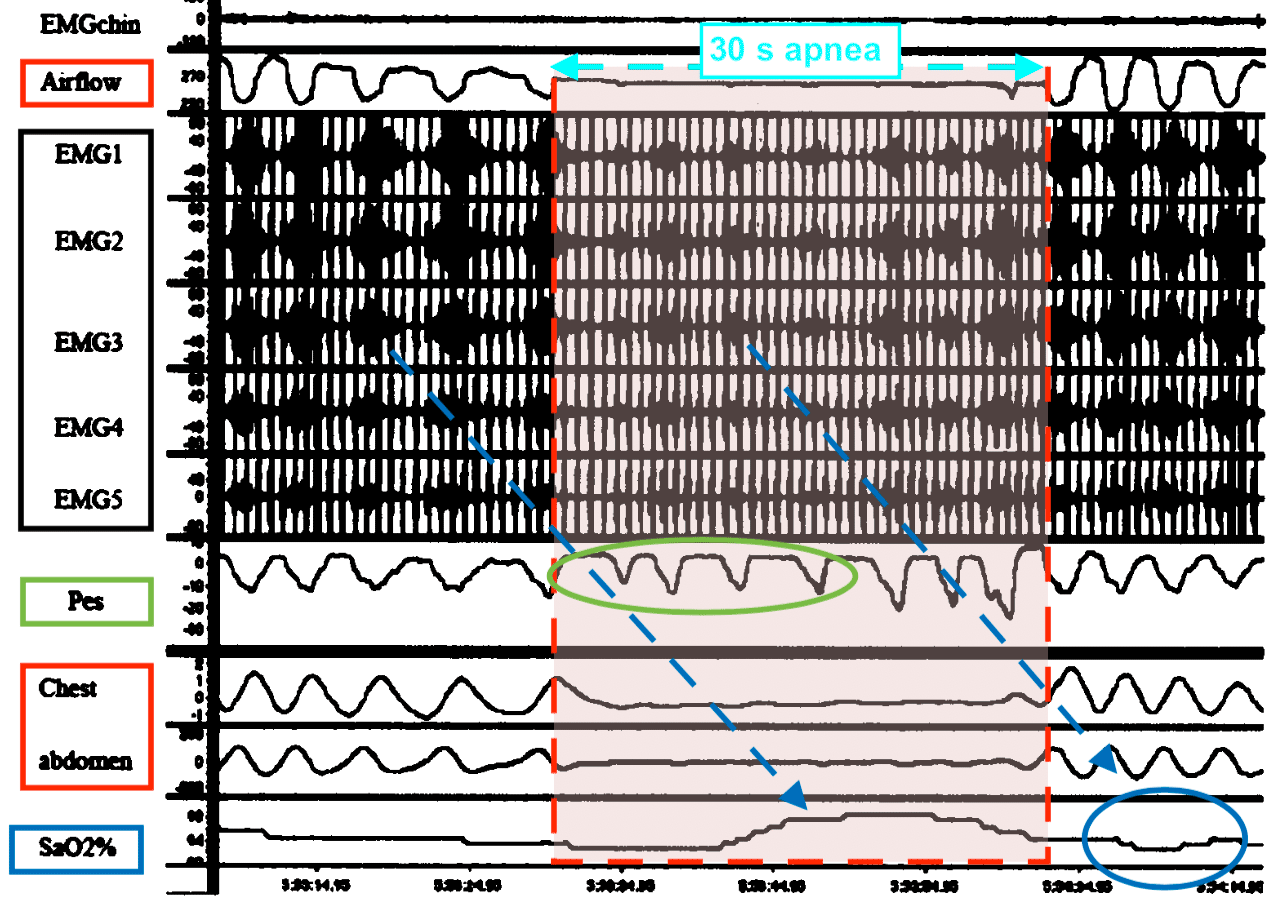
4. In an EMG study of an infant with a HE/cyanotic squirming episode [19] (Fig. 3), although diaphragm activity was not measured (like Fig. 1 above), surface abdominal EMG was used. It demonstrated increased muscle activity for 50 s that disrupted normal (tidal) breathing. A sustained elevation of esophageal pressure also occurred and was thought to be due to abdominal muscle contractions (perhaps voluntary). We felt spontaneous diaphragm contractions could have contributed to this, given the electrical activity could easily cross-contaminate the surface EMG. This made more sense given the numerous ventilatory issues observed and especially because this was a very young, weak and likely malnourished preterm infant, again highly unlikely to contract their abdominal muscles for such an extended period and to disrupt breathing to such an extent as to cause cyanosis. Interestingly, the episode was silent too (no crying), consistent with diaphragm arrest.
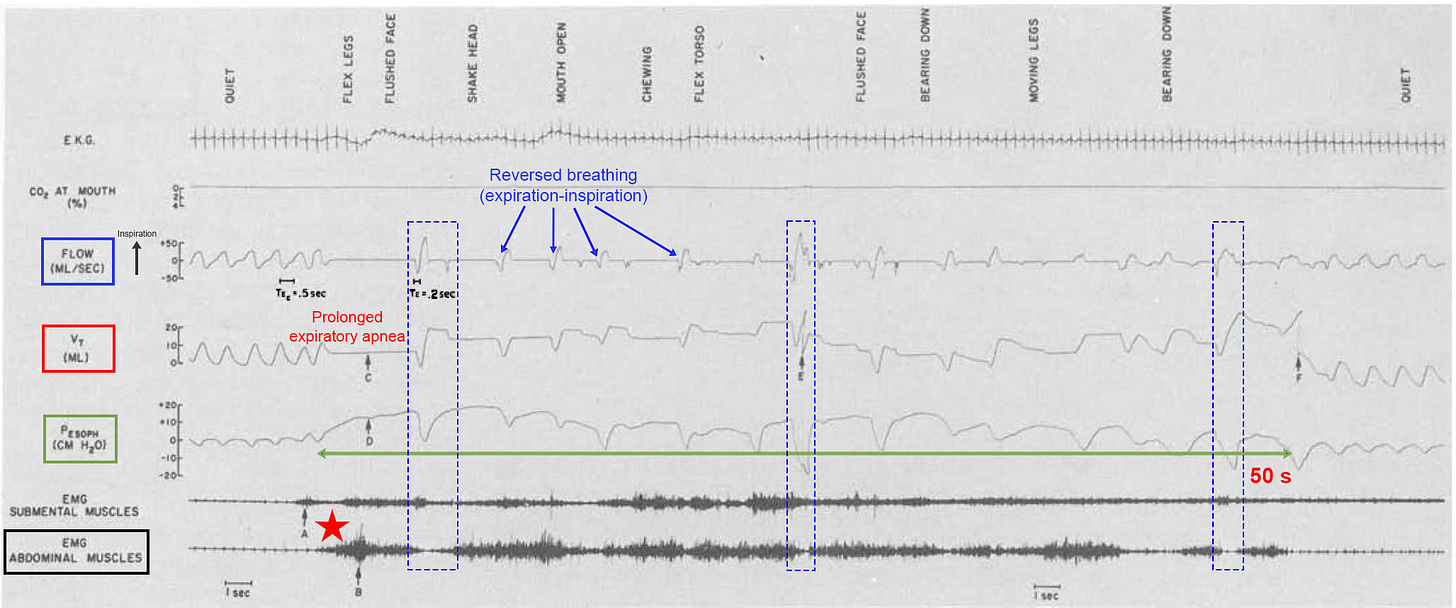
Given this new mechanism of sleep apneas (peripheral malfunction of inspiratory muscles), we went on to suggest that classic sleep studies (employing airflow and chest impedance, CI, measurements) may be interpreted differently:
Central apneas: Instead of failure of neural breathing, it is simultaneous failure of RAM and diaphragm by REM sleep and diaphragm spasm, respectively.
Obstructive apneas: Instead of the diaphragm breathing against upper airway obstruction, it is RAM against the diaphragm briefly inactivated by DS.
Mixed apneas: Instead of combined central and obstructive apneas, these occur when one of the inspiratory muscle groups “comes online” over the course of an apnea. For example, RAM activation by arousal during a DS. Or DS offset with functioning RAM.
Returning to the Patient 0’s bearhug apnea, he could not inspire despite trying. Had respiratory flow and CI monitoring been used, it would have appeared as a central apnea because of lack of chest expansion. Yet, there was complete airway obstruction, and it was probably caused by co-cramping of diaphragm and intercostal muscles. This is not the case in SIDS, however.
Diaphragm Failure in Sudden Unexpected Deaths
Hypothesis: Whereas transient DS induces brief apneas with hypoxemic episodes (which are survivable), persistent contractions, as DCC, cause sustained apneas (respiratory arrests) leading to life-threatening critical hypoxemia. Cardiac arrest would occur in only 1-2 minutes. Moreover, triggered by REM sleep or prone positioning, fatigue-induced DCC would cascade in a nocturnal, silent and rapid respiratory arrest death consistent with SIDS.
Hypothesis: Sleep DCC also occurs in older children and adults leading to nocturnal silent deaths consistent with SUDC and SCD, respectively. However, these are less common than SIDS because of mature respiratory muscles, whereby the threshold for critical diaphragm fatigue is higher.
Theoretically, for apnea/respiratory arrest to occur, there must be either: (1) a lack of neural stimulation (central apnea), (2) a complete airway obstruction (obstructive apnea) or (3) simultaneous failure of both diaphragm and RAM (peripheral apnea).
Using classical chest impedance measurements in a sleep study (sticky leads on chest & abdomen), the following have been interpreted historically:
“Central” apneas = no airflow + no respiratory movements of chest/abdomen.
Obstructive apneas = no airflow + respiratory movements of chest/abdomen.
Hypopneas (obstructed breaths) = reduced airflow + reduced movements of chest/abdomen.
1. The diagnosis of a central apnea assumes the lack of airflow and movements are caused by no neural input to the diaphragm. It was inferred, not actually measured (because that would require surgical implantation of an electrode in the neck to measure phrenic nerve activity). Unfortunately, this line of reasoning has perpetuated throughout mainstream research for many years. Peripheral failure (of diaphragm and RAM) was not taken into consideration. However, when Luo et al. compared CI to diaphragm EMG, many central apneas were actually peripheral [27]. Thus, true central apneas may not be as common as thought.
2. In addition, fatal spontaneous airway obstructions during sleep too have been called into question. The most commonly cited causes include laryngospasm (vocal cord airway closures) and atonic airway dilator muscles. In our opinion, both are insufficient to cause agonal breathing, critical hypoxemia, cardiac arrest and death because they are either transitory or incomplete, respectively, or both. Also, Southall demonstrated in nine children under 22 months’ age that severe HE still occurred despite endotracheal and tracheostomy tubes keeping the airway free of obstruction [17]. Taken together, this casted serious doubt about spontaneous airway obstructions causing fatal respiratory arrests, leaving the third option.
3. Peripheral apnea respiratory failure presents a lesser-known mechanism; yet a non-spontaneous version has already been discussed (i.e. acute diaphragm paralysis from nicotine overdoses, succinylcholine or envenomations). Both diaphragm and RAM must fail simultaneously for this form of apnea to occur, and it appears to result by a variety of mechanisms:
In Patient 0 with complete respiratory arrest (“like a total airway obstruction”), we proposed there was simultaneous failure of diaphragm and RAM: (a) DCC and (b) cramping of ICM (co-cramping).
In sleeping infants with diaphragm excitation triggered by REM sleep RAM inactivation, sustained respiratory arrest would require the infant does not wake up to reactivate RAM. Impaired arousal has been considered a SIDS risk factor, so could explain this. However…
While the first two possibilities could cause respiratory arrest, they do not explain SIDS autopsy findings (i.e. evidence of obstruction). Something needed to provide inspiratory force (to generate the negative intrathoracic pressures) and also breathing against some form of reversible airway resistance.
This could be explained by a combination of DCC with ineffective RAM contractions, due to their inherently weak state in young infancy (i.e. underdeveloped and untrained). Like the EMG inspiratory mechanical failure shown in Fig. 2 above, RAM are hereby proposed to be semi-functional. Their action would generate inspiratory efforts and create negative intrathoracic pressures yet fail to expand the lungs with air (ineffective gasping).
Hypothesis: Like the hypercontracted, incapacitated diaphragm of DS mentioned above (causing brief apneas in OSA), DCC itself is the mysterious airway obstruction in SIDS. It would resist agonal RAM pumping actions, thus explaining autopsy findings. It would also disappear with postmortem relaxation (and is why DCC has evaded detection all this time).
The following two slides provide a summary of the proposed SIDS pathogenesis based on the novel diaphragm pathology presented here.
Male Preponderance in Respiratory Instability and SIDS
The male sex is a well-known SIDS risk factor, as 60% of victims are male (ratio of 1.5:1). This is an extension to the “male disadvantage” seen in respiratory morbidity and mortality in early life. Compared to preterm girls, preterm boys have more frequent and more severe HE along with its host of short- and long-term complications, including death from respiratory causes [23,28]. Interestingly, there is also a male preponderance to cerebral palsy, ADD/ ADHD and autism29, suggesting a common mechanism (which we think is recurrent HE from repeated DS in early infancy).
Hypothesis: Compared to female infants, male diaphragms are more susceptible to fatigue and work overload. This culminates in recurrent HE from pathological diaphragm excitation and is responsible for the male RI and SIDS gender gap. It also contributes to the increased likelihoods of cerebral palsy, ADD/ADHD and autism seen in males.
The overall supporting evidence for fatigue-prone diaphragms in males was compelling. Compared to females, males had thinner diaphragms on ultrasound (weaker and forced to work harder*), elevated total airway resistances (causing higher ventilatory workloads), reduced respiratory exercise endurances as well as less efficient diaphragm contractions and ICM recruitment. Also, male mice exposed to in-utero (birth) asphyxia had lower survival rates at one hour after birth compared to females (52% versus 69%, respectively) [22]. Their diaphragms had significantly worse structural and functional deficits, suggesting that hypoxemia-induced, gender-skewed diaphragm failure had caused the deaths. (*In that ultrasound study, the authors suggested the reduced diaphragm thickness translated to diaphragm weakness30.)
Unsurprisingly, adult males too have a higher incidence of OSA than females. Night sweats, also more common in men, could reflect the added work of breathing by a fatigue-prone diaphragm, especially in REM.
Another surprise though was a male preponderance of leg (calf) muscle inflammation (myositis) and damage (myopathy) in children and adults with influenza31. [Perhaps the diaphragm too could become involved in these viral infections (viral myositis/myopathy)?]
Viral-induced diaphragm contractile dysfunction (myopathic weakness), worse in infant males, combined with added resistance from upper airway and pulmonary congestion in infection, could increase diaphragm workload, fatigue and potential for spontaneous DCC leading to SIDS. This would explain the male SIDS predominance.
Diaphragm Injury and Congested Organs at SIDS Autopsy
The strongest support for DCC in SIDS came from a systematic diaphragm autopsy study by Kariks (1989)32. Although macroscopic (gross) abnormalities were not seen, microscopy with special staining (histology) revealed myofiber ruptures and diaphragm contraction band necrosis (CBN) in 198 of 242 SIDS cases (82%), along with fibrotic scars. The CBN had occurred rapidly and terminally under severe hypoxia (anoxia), close in time to the cardiac arrest. Despite a few other reports confirming these myopathic changes in SIDS, the line of research went inexplicably silent.
Diaphragmatic CBN: has also been reported in deaths from birth asphyxia (the most common category) as well as those from perinatal and infantile infections (second most common), including respiratory, gastrointestinal, bacterial sepsis and meningitis. Other deaths included motor vehicle accidents, anesthetics, drownings, asthma and carbon monoxide exposures (house fires and burns). Proposed causes of CBN included hypoxia-ischemia, viral myositis and metabolic mismatch (fatigue) with excess catecholamines [epinephrine (adrenaline) and other adrenal hormones].
The CBN-associated deaths from a variety of causes in children suggest a common mechanism. DCC could represent the terminal mechanism in all.
Diaphragm myofiber ruptures: Dr. Michael Eisenhut MD published an important report in 2011 [who later became a co-author of the original DCC paper]. Tragically, a 5-month-old previously healthy girl, who was admitted for viral respiratory infection (rhinovirus) and poor feeding (adenovirus), had died in hospital by a sudden unexpected respiratory arrest. Diaphragm histology at autopsy revealed myofiber destruction, necrosis and regeneration as well as inflammation33.
Both abnormalities have been reported in “clinically unexplained” sudden deaths in children and adults with viral infections (e.g. RSV, influenza, coxsackievirus and COVID-19 [13,34]). They demonstrated that viral-associated respiratory arrests can occur suddenly, rapidly and unexpectedly in all ages. This is consistent with myopathy-induced DCC.
In addition to viral myopathy, a clue to the origin comes from experimental loading of rabbit inspiratory muscles well above their fatigue thresholds35. In other words, breathing against a near-total airway obstruction (resistive breathing). Myofiber disruptions and significantly inflamed necrotic tissues were demonstrated in all test animals. Moreover, they occurred in a load-dependent manner whereby higher ventilatory workloads correlated to larger areas of damage (myopathy). There was no mention of D-CBN, however, none of the rabbits had died during testing (presumably no DCC). Myofiber disruptions (but not necrosis or inflammation) were also seen in 29 of 29 human test subjects exposed to less severe inspiratory loading36.
Hypothesis: The airway obstruction of DS/DCC could be the source of the diaphragm myofiber disruptions seen in SIDS. With continued obstructed breathing under severe hypoxemia, necrosis would develop (as CBN).
Organ weights in SIDS are significantly higher than normal, including the brain, lungs, thymus, liver and spleen3738. Negative intrathoracic pressures (nITP) generated by breathing against obstruction partly explains the heavy, wet lungs because of blood pooling (shunting) into the pulmonary circulation (see Pulmonary System below).
To explain this from a diaphragmatic perspective, we considered its 3-dimensional anatomy. Specifically, that the inferior vena cava (IVC) and aorta pass through its left-to-right axis in the perpendicular plane (up and down). They can be seen in cross section in Fig. 4 below as they course through their diaphragmatic apertures (hiatuses or foraminae), passing from abdomen to chest and vice versa, respectively. The IVC — a major vein carrying deoxygenated blood away from the abdominal organs and lower extremities to the heart — and the aorta (artery transporting oxygenated blood away from the heart and lungs to all organs and extremities), are the two largest blood vessels in the body.
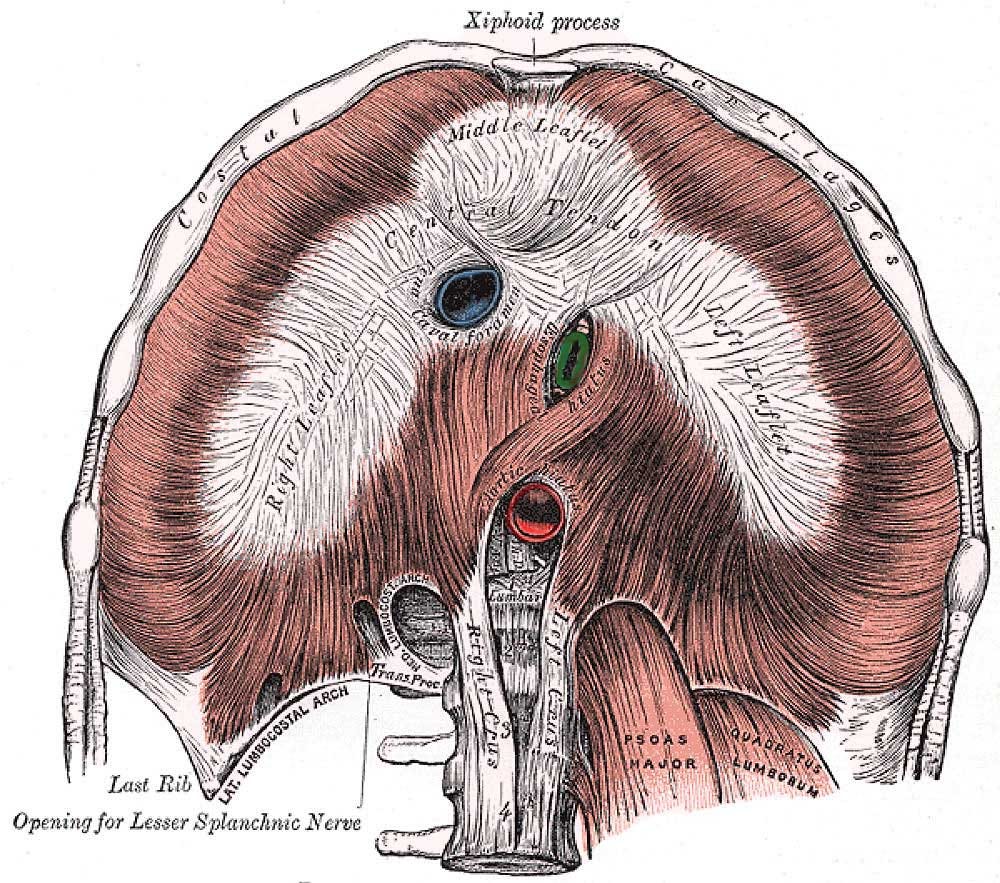
In diaphragm excitation, the squeezing force of DS/DCC could partially clamp (occlude) these great vessels, leading to reduced blood flow above and below the diaphragm, but to different degrees depending on location of the organ or extremity in question and position relative to the heart. Also, cardiac output, blood pressure and venous drainage would simultaneously be affected, all leading to reduced organ perfusion and injury.
Aortic clamping at the diaphragm could induce an immediate hypertensive spike in organs and extremities above the diaphragm. Those below would likely face hypotension.
The effects of IVC clamping on blood pressure would take longer to appear as venous congestion develops gradually. Over time, less and less blood returns to the heart, worsened by nITP-pulmonary shunting. Ultimately, the combination of persistent IVC and aortic clamping and nITP in diaphragm cramp would eventually induce severe systemic hypotension (shock).
The pulmonary system presents a unique hemodynamic situation in DS/DCC because of its blood supply from the superior and inferior vena cava via right heart. In addition, this circuit is affected by intrathoracic pressure. Normally during inspiration, blood is transiently shunted to this circuit with no untoward effects. However, with obstructed inspirations, severe negative pressure surges develop. Pulmonary vascular pooling and pulmonary hypertension (PH) occur, leaving less blood for the left heart to pump (thus, even more hypotension and reduced organ perfusion). Pulmonary edema (fluid in the alveolar air spaces) and vascular congestion (engorged pulmonary vessels) would both develop if DS persists (as DCC), potentially explaining the wet, heavy lungs seen at autopsy in SIDS.
Esophageal Clamping by Diaphragm Excitation
The other organ that traverses the diaphragm is the esophagus. It too can become effectively squeezed or completely occluded by diaphragm hypertonicity or excitation. Consequent gastrointestinal symptoms could arise. Indeed, reports of diaphragm flutter revealed epigastric (and chest) pain and pulsations, hiccups, belching, retching, nausea/vomiting and GERD. Interestingly, there is an increased incidence of GERD in preterm infants with HE, as well as in adults with sleep apnea. In the latter, symptoms improved with CPAP (unexplained). This could have occurred because CPAP reduces diaphragmatic workload. Similar gastrointestinal symptoms were also reported in children with focal seizures39. Perhaps diaphragm hyperstimulation was responsible (see DCC in Seizure Deaths below).
“Stomach butterflies”, the commonly experienced anxiety-provoked sensation of a transient wave-like motion in the upper abdomen, could be due to diaphragm excitation. This would suggest a connection between mind and body at the diaphragm.
At autopsy in SIDS, SUDC and SUDEP, stomach contents are sometimes found in the upper airways and mouth. Postmortem release of the putative esophageal clamp could be responsible. As we know from autopsies demonstrating agonal obstruction (i.e. evidence of severe nITP) as well as the inverse chest/abdominal pressure relationship, then there must have been equally elevated intra-abdominal pressures providing the force behind gastric expulsion.
Compression of IVC and Aorta by Diaphragm Spasm
In a baby with a 60 s cyanotic HE seen in Fig. 5 below (cyan ellipse) marked by a sudden change in respirations (blue rectangle), there was a rise in arterial blood (systemic) pressure (black ellipse and arrow) [17]. Although D-EMG and gastric pressures were not available to confirm it, we hypothesized DS was responsible, by compression of the aorta at its diaphragmatic hiatus (causing increasing vascular resistance). With simultaneous nITP surges from DS airway obstruction, both caused the pulmonary blood pressure to increase as well (pulmonary hypertension, PH, green ellipse). Because the entire event was brief and the baby survived (oxygen saturations returned to normal), DS was favored over DCC. Had excitation lasted longer, as DCC, we believe critical hypoxemia, hypotension and cardiac arrest would have occurred (consistent with SIDS).
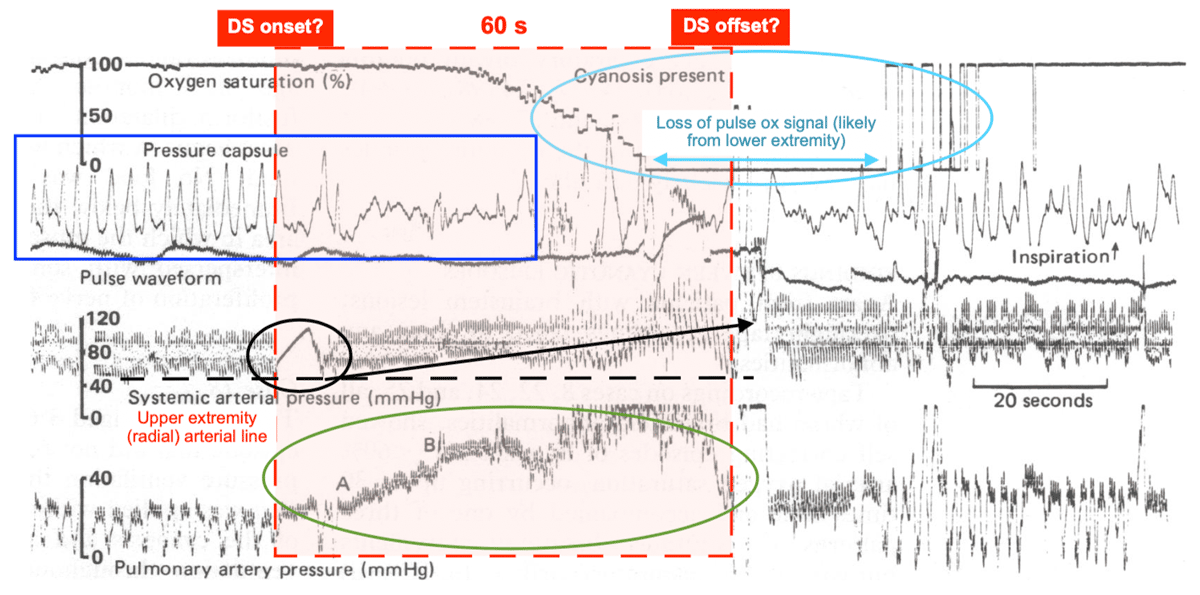
In this same study of 51 children with cyanotic HE, 76% were otherwise healthy and most outgrew them, but 8 (16%) died suddenly and unexpectedly,“consistent with SIDS” (per authors) [17].
D-EMG and gastric pressure changes associated with a HE were reported by the same group in Fig. 6.40 It demonstrated how bursts of abnormal diaphragm contractions are associated with time-equivalent esophageal pressure changes and abnormal grunting ventilations (along with tachycardia, systemic hypertension, mild oxygen desaturations). Again, it is important to note that although hypertension occurred during this brief HE, hypotension and shock would likely have developed if contractions were sustained.
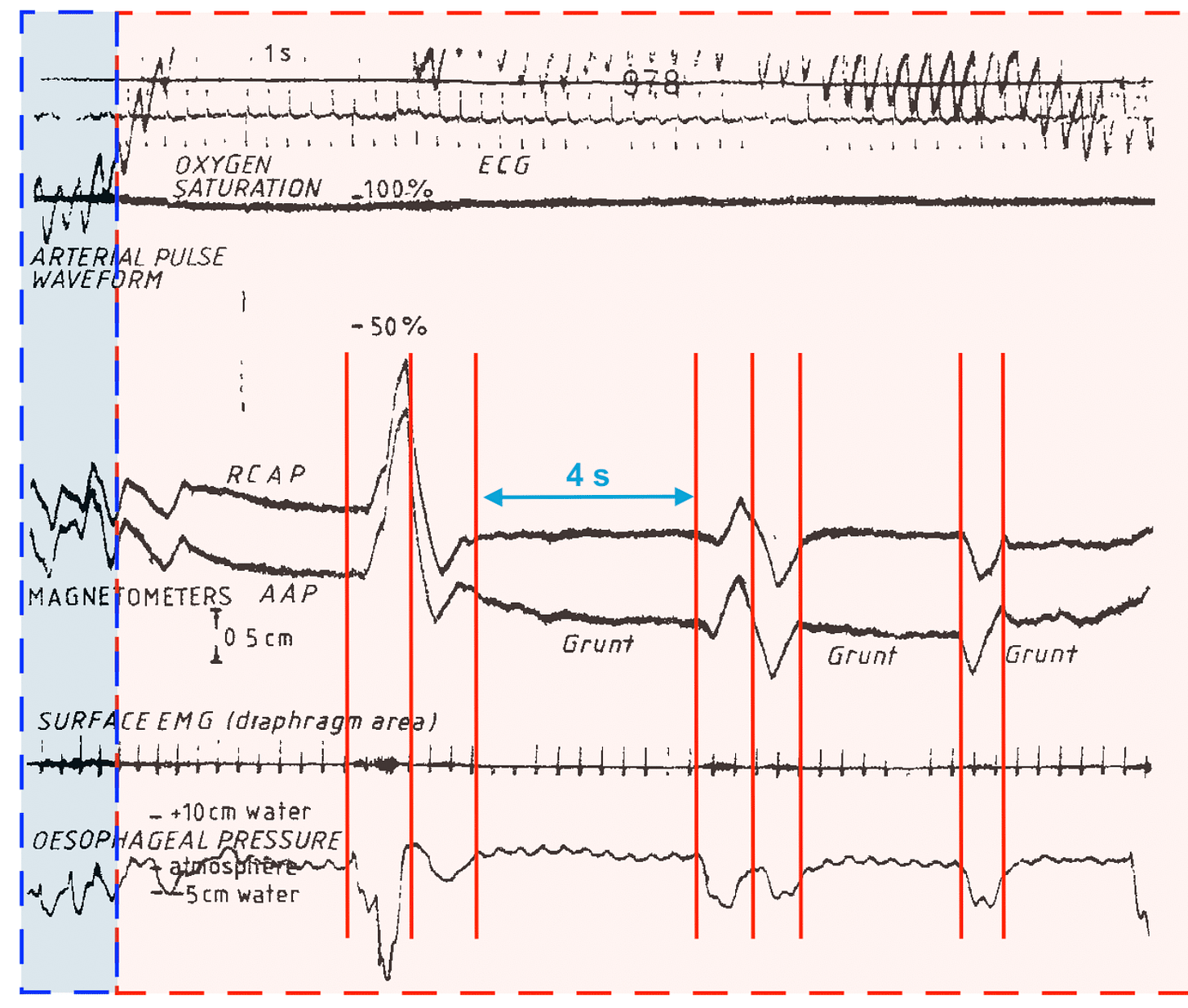
New grunting and short respiratory pauses (apneas) in a young infant may indicate diaphragm spasms and imminent respiratory failure (especially in males and if ill). The baby should be seen by a doctor immediately.
Complications of Repeated Hypoxemic Episodes from Diaphragm Spasms
Repeated sleep-DS-induced hypotension with venous congestion and HE might be responsible for many complications of prematurity, including:
Bowel ischemia, infections and perforations (necrotizing enterocolitis)
Progressive hypoxic-ischemic damage to the small, fragile blood vessels of the eyes (retinopathy and visual impairments)
Progressive injuries to the brain (periventricular leukomalacia, white matter injury and intraventricular hemorrhages)
Cerebral palsy41, ADD/ADHD [24] and autism42, all of which are associated with perinatal brain hypoperfusion and hypoxic injury [Decima 2015]
Recurrent DS-HE in children and adults with RI and OSA, respectively, are proposed to be triggered several times each night by REM sleep and occur over a lifetime if undiagnosed. Although the brief hypoxemia and hemodynamic abnormalities may be tolerated acutely, cumulative strain and end-organ damage may occur over the long term, especially the heart, lungs and brain.
For example:
Recurrent episodic blood pressure surges from PH in young children would strain the heart regularly, leading to reversible wall dilatations and valve strain at first. By later childhood, continued episodes would cause right-sided cardiomyopathies (e.g. dilated, hypertrophic) and chronic PH.
If continued into adulthood, untreated OSA would lead to hypertension, congestive heart failure and coronary artery disease (heart attacks) amongst other cardiovascular disorders. This combination also increases the risk for heart arrhythmias, some of which can be malignant, causing sudden unexpected “cardiac” deaths.
Recurrent episodes of reduced venous drainage of the legs would increase the risk for deep blood clots, some of which can travel to the lungs resulting in pulmonary embolism, an emergency.
All these conditions are more common in those with OSA.
Table 3 shows the complete spectrum of complications from DHD across all ages. This includes those of RI in infants, and OSA in older children and adults, but also sudden unexpected deaths.
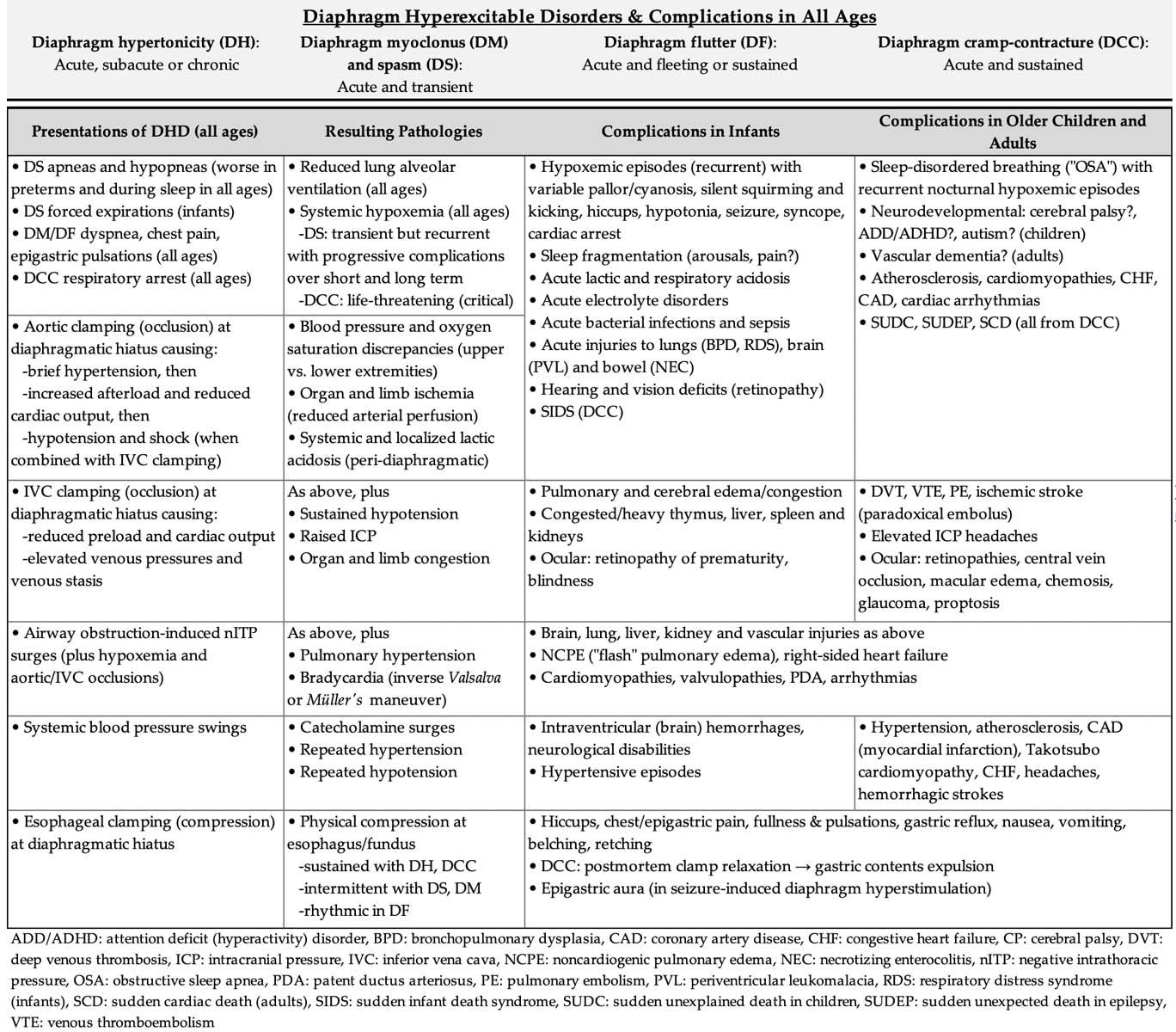
DCC in Seizure Deaths (SUDEP)
SUDEP refers to a sudden unexpected death of a person with epilepsy with no clear cause found at autopsy. It typically occurs during sleep or shortly after a seizure. Although it can happen at any age, young adults aged 20-40 are affected most commonly, demonstrating a male preponderance.
There are many shared autopsy findings between SIDS, SUDC and SUDEP, suggesting a common mechanism. We believe it to be DCC, however, the pathological sequence of events in seizure leading up to diaphragm failure differs to those already discussed.
Seizure activity from the CNS may be transmitted to the diaphragm by the phrenic nerve(s), causing diaphragm hyperstimulation and subsequent fatigue. These excessive contractions could induce DCC respiratory arrest either immediately or in delayed fashion, after a brief period of escalating lactic acidosis and hypoxemia. This may contribute to critical diaphragm fatigue, whereby postictal REM sleep onset, tachypnea or even a body roll to prone position suddenly triggers DCC.
Best supporting evidence for DCC in SUDEP came from three sources:
1. In forensic autopsies comparing 13 SUDEP cases and 31 non-SUDEP sudden deaths in those with a history of epilepsy, the primary mechanism of death was asphyxia in the SUDEP group versus cardiopulmonary failure in non-SUDEP43. The SUDEP group was younger, more cases had an uncertain etiology of epilepsy* and were found in prone position. They also had more cardiac lesions and pulmonary congestion and edema at death. We believed that fatal DCC with obstruction was responsible. [*Perhaps DS hypoxemia is an unrecognized seizure mechanism in epilepsy.]
2. In 100 children with 514 videotaped focal seizures, mostly stemming from the temporal lobe which is involved in volitional (nonspontaneous) breathing, a multitude of periictal respiratory and gastrointestinal symptoms were experienced. These included hyperpneas, apneas and bradypneas as well as hiccups, belching, nausea/vomiting and “epigastric aura” [39]. Epigastric (or visceral) aura is a symptom complex of short duration involving ictal abdominal discomfort, nausea and/or a burning sensation. It occurs most often with temporal lobe seizures and receives unusual descriptions such as “fluttering, pressure and rolling or turning of internal organs”. The gastrointestinal symptoms could all be explained by the physical effect of seizure-induced diaphragm tone/contractions on the esophagus/stomach, whereas the respiratory symptoms from diaphragm tone/contractions, overload, excitation and delayed resetting (causing bradypneas).
3. Using D-EMG in mice, experimental seizures induced immediate respiratory arrests (terminal apneas) by sustained (tonic) periictal diaphragm contractions44. Deaths occurred not immediately (not periictal), but rather when the apnea persisted into the postictal period.
Abdominal Winding Injuries and Diaphragm Excitation
This has partly been discussed above. In addition, like the tympanic membrane of the ear, the diaphragm serves as a hermetic seal between two anatomic compartments held under different air pressures (chest and abdominal cavities) (Fig. 7). A pressure increase in one causes a decrease in the other, and vice-versa, with the diaphragm buffering between extremes.
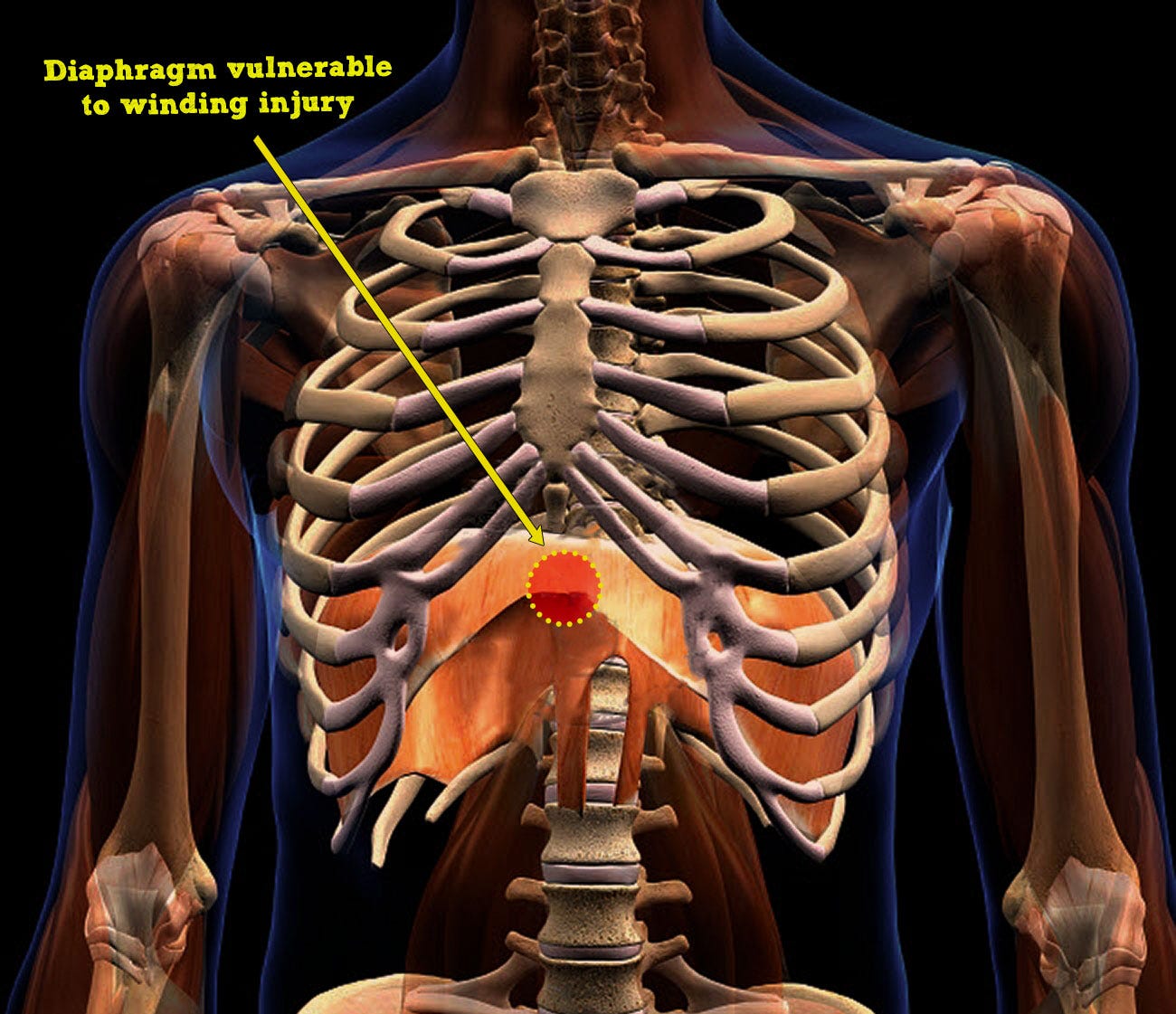
The tympanic membrane can tear from extreme air pressure changes, like SCUBA diving barotrauma. Similarly, the diaphragm can rupture from significant internal air pressure changes caused by high velocity impacts to the abdomen or chest, typically, motor vehicle collisions and falls from significant heights.
Being winded (celiac or solar plexus syndrome) occurs by a relatively low velocity, non-penetrating blow to the epigastrium/chest, causing pain, forced expiration and apnea. For example, from bodily collisions, a punch or kick to the epigastrium (or blow by a hockey stick or baseball bat) or perhaps a slip and fall onto the back. Anyone who has experienced this has probably learned to protect themselves from reexperiencing the duress, by flexing their abdominal muscles in anticipation of a strike (to absorb some of the force). Also, instead of the widely held notion of celiac plexus stimulation causing symptoms, it is rather thought to be primary (i.e. organ automaticity) given the posterior location of the plexus, protected from impacts.
The kinetic force of low velocity blunt abdominal/chest trauma is transmitted to the diaphragm, triggering neuromuscular excitation in the form of brief spasms (“traumatic DS”, t-DS) or prolonged cramp (“traumatic DCC”, t-DCC). The latter occurs in higher energy impacts. Apnea occurs in both, and duration dictates symptoms and survivability based on degree of subsequent hypoxemia and potential for cardiac arrest. Syncope and collapse may precede cardiac arrest (Damar Hamlin collapse). If death occurs, it would be ruled a “traumatic cardiac arrest”, thus missing the primary respiratory etiology.
The concern in t-DS is not the pain nor involuntary forced expiration, but the involuntary apnea that persists for an uncomfortable few seconds. Unfortunately, although t-DS has been reported in the literature to be the mechanism, supporting evidence was not provided [9]. Evidence for the t-DCC mechanism was unavailable too, primarily because of a lack of experimental studies and inherent limitations of observational case reports in fatal cases [Maron et al. 1995]. Again, because of the reversible nature of diaphragm excitation, it does not subsist at autopsy. As well, many sudden deaths (and football player collapses) from blunt bodily impacts have been classified as commotio cordis despite lacking two important criteria needed to establish this diagnosis.
Commotio cordis (CC) is defined as sudden cardiac arrest resulting from a non-penetrating impact to the chest wall (which overlaps with t-DS/DCC), but typically by a projectile like a hockey puck or baseball. When timed to a specific phase of the cardiac cycle (during relaxation), it can induce a fatal ventricular arrhythmia: ventricular fibrillation (VFib) [or ventricular tachycardia (VT)], which is the ultimate cause of the collapse. However, in the defining experimental animal models of CC, it was reproduced by a focal blow over the heart and no other chest region [Link et al. (1998)].
Not all cardiopulmonary arrests from bodily impacts are due to commotio cordis. They may also be caused by t-DCC respiratory arrest, which might have been the case in the Damar Hamlin collapse.
As opposed to the focal aspect of CC, non-focal (blunt) impacts to the chest or epigastrium causing t-DCC respiratory arrest can also induce syncope/collapse and death (by secondary cardiac arrest rather than primary cardiac arrest). Severe hypoxemia may ultimately lead to the same ventricular arrhythmias seen in CC (VFib or VT), thus mimicking CC. However, there is an important difference, and this translates to prognosis…
CPR priorities differ in collapses from CC and t-DCC because of divergent primary issues:
t-DCC respiratory arrest: Airway opening and rescue Breaths (A-B)
CC cardiac arrest: Chest compressions and Defibrillation (C-D)
This might improve the currently dismal success rate of CPR in traumatic arrests.
Poor survival (and outcome) occurs in collapses from CC, historically, as low as 10% despite immediately available life-saving measures (e.g. paramedic CPR at competitive sporting events, bystander CPR at others). Although survival has improved since the 1990s, perhaps due to increased availability of portable automated external defibrillators allowing more time to get to the ER, the poor survival could be due to refractory VFib, coronary artery vasospasm, myocardial contusion or inadequate management of the patient’s airway and breathing.
It is also important to note that the respiratory arrest of t-DCC would not be obvious to bystanders because of complete airway obstruction. Inspiration does not occur and so it would be silent.
An issue constantly plaguing bystander CPR is the lack of will to initiate mouth-to-mouth rescue breaths (fear of communicable infections, particularly so when the victim is not a child). Also, even if they are attempted, most are done incorrectly (needs a seal and to ensure chest rise with each rescue breath). In addition, this important recommendation apparently went missing since the arrival of the 2005 CPR guidelines.
As such, it may only be once the victim is in the ER when the airway is finally managed properly. But even then, the added airway resistance of DCC requires harder squeezing of bag-mask ventilations, something feared by healthcare providers because of potential lung overinflation injury.
Reports of added airway resistance on initial ventilatory efforts were made by Southall and others, as well as this writer’s experience as an ER physician. It may have been due to the hypercontracted state of DS/DCC. If higher bagging forces are initially used to overcome it, survivability may improve.
Improved survival in traumatic and spontaneous DCC cardiopulmonary arrests may occur with improved rescuer management of the airway and breathing (by both lay public and healthcare provider). This involves overcoming the added airway resistance initially and then visually confirming chest rise with each rescue breath delivered.
Diagnosing Diaphragm Fatigue and Excitation
From frequent hiccups and RI in neonates (i.e. apneas-hypopneas, periodic breathing, HE) to bronchiolitis, apneas, breath-holding spells and life-threatening symptoms in older babies and young children, the various etiologies and parameters of underlying diaphragm fatigue and excitation can be evaluated.
Objective signs of diaphragm fatigue are the same as those of “respiratory insufficiency”, a term commonly used in the emergency room to describe ventilatory distress. But instead of focusing just on the lungs, consideration should also be made to the respiratory muscles. Signs in infants include labored breathing, tachypnea, nasal flaring, grunting, wheezing, sweating, pallor/cyanosis, rib retractions and hiccups. Sleepiness and paradoxic breathing are very serious findings. Signs in adults are similar, including preference for sitting upright and sudden confusion or sleepiness.
Signs of DS and DF overlap, however, some are specific to flutter. DS and DF: recurrent or prolonged apneas, desaturations, tachypnea, hypoventilations, bradycardia, rib retractions, paradoxic breathing, cyanosis, pallor, hypotonia, syncope, seizure, unexplained events, frequent hiccups. DF: epigastric pulsations, ratchet-like inspirations and fluttering, murmur asynchronous with heart, friction rub, sense of impending doom.
ECG: Sinus tachycardia, bradycardia, intermittent ST repolarization abnormalities, T-wave notches and inversions. Avoid dismissing artifacts as body movements; excitation could be responsible.
Lab testing (Table 4) can assess for the causes and outcomes of diaphragm fatigue and excitation, primarily DS and DF. The former includes anemia (a cause of life-threatening apneas and other RI in preterm infants) [Poets et al. 1992], magnesium deficiency and other electrolyte disorders [Caddell 2001] and abnormal blood acid-base balance (metabolic acidosis and alkalosis) [15]. The latter includes screening tests to reveal diaphragm damage from hypoxia, hyperthermia, myopathic viral infections and/or resistive loading include serum creatine kinase (CK or CK-MM, muscle type) and skeletal muscle troponin-I (STnI). Although not specific to respiratory muscles, correlating levels to changes in clinical state would be helpful. In infants with bronchiolitis, high CK levels could screen those at higher risk for respiratory distress and sudden apneas. This alone would not only save lives from SIDS but also reduce the flu/RSV season annual burden on health care systems, translating to reduced costs and waiting times (and satisfied parents).
Suggested Labs Tests (screening, trends and respiratory distress PRN):
-Nasopharyngeal swab (r/o respiratory viruses)
-Hemoglobin (r/o anemia)
-BUN/creatinine ratio, serum osmolality (r/o hypovolemia)
-Extended electrolytes (sodium, potassium, calcium, magnesium, phosphate)
-Lactate and VBG, including pH, pCO2, HCO3 (r/o hypercapnic failure)
-Nicotine and metabolites (r/o absorption from tobacco smoke).
-CK-MM or sTnI (correlate with VBG and respiratory distress PRN)
PRN: Pro re nata (as necessary), r/o: rule out, VBG: venous blood gas, CK-MM: creatine kinase muscle fraction, sTnI: fast isoform skeletal muscle troponin-I. CK-MM may be elevated in DS, DF, RF, DCC and even from the hypertonicity of diaphragm fatigue.
Table 4: Proposed labs and biomarkers revealing evidence of diaphragm fatigue, excitation and myopathy.
Other investigations (Table 5) to evaluate for active diaphragm excitation (DS, DF, RF) include diaphragm ultrasound and EMG along with RIP. Together, these might determine if the diaphragm is contracting independently (automaticity) or by neural stimulation.
Suggested Investigations (monitoring and active excitation PRN, histology):
Continuous RIP and transcutaneous/esophageal D-EMG in high-risk individuals*
Bedside abdominal US and D-CEUS (perfusion) in suspected active DHD†
4D CT (rapid sequence of images) in suspected active DHD†
Cine MRI (rapid sequence of images) in suspected active DHD†
Bedside fluoroscopy (C-arm) or dynamic chest radiography in active DHD†
Diaphragm biopsy (r/o myofiber disruptions, inflammation, contraction bands, fibrous scars)
RIP: respiratory inductive plethysmography, D-EMG: diaphragm electromyography, US: ultrasound, D-CEUS: diaphragm contrast-enhanced ultrasound, DHD: diaphragm hyperexcitation disorders († DS, DF, RF or near-DCC). * Those in respiratory distress or have lab abnormality or SIDS sibling(s).
Table 5: Proposed monitoring and imaging revealing evidence of diaphragm fatigue, excitation and myopathy.
Treatment Options
Table 6 provides emergent interventions and potential medications identified on preliminary literature review that might improve diaphragm fatigue and thus prevent or attenuate excitation. In patients of any age with respiratory distress, this includes correcting anemia, electrolyte disorders and acidosis as well as providing supplemental oxygen, rehydration and antipyretics as needed.
Methylxanthines such as caffeine and theophylline have been used for over 50 years to reduce apneas-hypopneas and HE in infants. They are thought to stimulate the CNS respiratory centers; however, also have peripheral effects on respiratory muscles. Chlorpromazine, an older antipsychotic with relaxation effects on the CNS and skeletal muscles, was most commonly used to treat DHD, particularly effective in intractable hiccups and RF. Its calming effect might even reduce diaphragmatic hypertonicity in extreme anxiety. N-acetylcysteine has shown promise by improving diaphragm force generating capacity and anti-inflammatory activity in a mouse model of muscular dystrophy. Vitamin E, an antioxidant, reduced muscle fiber damage, oxidative stress and inflammation in test animals. Creatine, a high-energy phosphate source in muscle, enhanced protein synthesis and muscle function. Finally, diaphragm pacing might treat or prevent unstable DHD.
Treatment is a high priority in OSA because of its ubiquity and very serious comorbidities. However, despite the use of CPAP improving OSA severity in adults, long-term benefits such as reduced hypertension were minimal. Instituting therapies tailored to reducing respiratory fatigue and workload in OSA offers a novel approach.
Emergent Interventions for Diaphragm Distress
Supplemental oxygen
Intravenous access
Cardiac monitor, RIP, pulse oximetry, capnometry
CPAP, nasal mask or intubation/MV
Minimize psychiatric stressors and pain
Stop antireflux medications
Anticonvulsant medications PRN seizure
Treat bronchospasm (β-agonists, theophylline?)
Correct hypovolemia and acidosis (bicarbonate?)
Correct anemia and electrolyte disorders
Treat infection (antibiotics, antivirals?)
Body cooling measures PRN
Diaphragm or phrenic nerve pacing?
Example Medications in Respiratory Distress from Diaphragm Dysfunction
Caffeine, theophylline
Chlorpromazine, haloperidol
Carbamazepine, phenytoin
Gabapentin, pregabalin
SSRIs (sertraline, citalopram, fluoxetine)
Cyclobenzaprine
Benzodiazepines
ACE inhibitors (lisinopril, ramipril, captopril)
Calcium channel blockers (verapamil, amlodipine, nifedipine, nicardipine)
β-agonists, sympathomimetics (salbutamol, clenbuterol, isoproterenol, procaterol)
Erythropoietin
N-acetylcysteine
Vitamin E (⍺-tocopherol)
Creatine
RIP: respiratory inductive plethysmography, CPAP: continuous positive airway pressure; PRN: pro re nata (as necessary); SSRIs: selective serotonin reuptake inhibitors, ACE: angiotensin-converting enzyme.
Table 6: Preliminary list of interventions and medications that may improve diaphragm fatigue and reduce subsequent HE. All medications were found on broad literature review and have not been tested or confirmed. They are here for demonstrative purposes only.
Conclusions
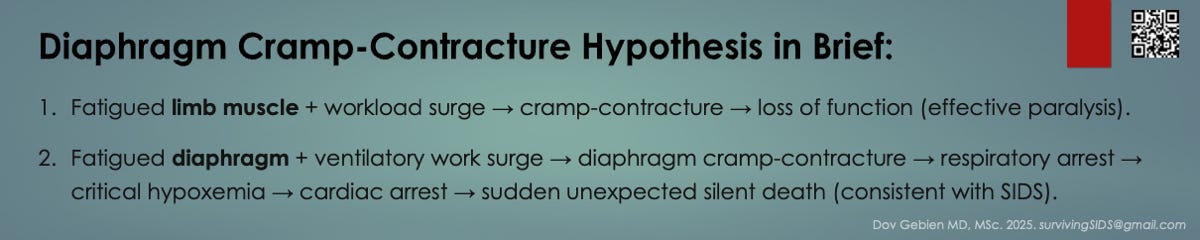
It is exceptional the wealth of new medical insights stemming from a solitary patient, let alone just two symptoms (bearhug apneas) that awoke him from sleep sporadically throughout his childhood and youth, starting at 7 years old. Each episode caused a near-death experience from a spontaneous inspiratory arrest involving previously under-appreciated or novel diaphragm pathologies.
Clinical reasoning using key historical features allowed us to reduce the differential diagnosis to putative diaphragm cramp-contracture (DCC), a completely new diagnosis. We hypothesized that spontaneous neuromuscular excitation of the diaphragm (cramp) had induced acute respiratory arrest (apnea by contracture). Like limb cramps, it is triggered by work overload of a fatigued muscle.
Diaphragm excitation by work overload during sleep is thought to primarily occur by REM sleep CNS inhibition of accessory inspiratory muscles (which normally share ventilatory workload with the diaphragm). Alternatively, it could be triggered by a body roll to prone position, airway obstruction or an increase in neural respiratory rate (from pain, stress, noxious stimuli, worsening infection and more). Excitation typically appears intermittently and briefly, and together with its unpredictability combined with internal location of the diaphragm make it extremely difficult to observe in sleep studies.
Surprisingly, aside from hiccups (which are generated by diaphragm contractions), a variety of other diaphragm hyperexcitability disorders (DHD) had already been reported in the literature, including diaphragm spasms, flutter and myoclonus. Spontaneous and traumatic forms of diaphragm spasm existed, both exhibiting forced expirations and involuntary apneas and hypopneas (obstructed breaths) in all ages. It appears when spasms are brief, hypoxemic episodes result, and the patient recovers because of its transience (subcritical hypoxemia). We added that if spasms are sustained (as DCC), critical hypoxemia ensues. Death would be rapid and silent (from inspiratory arrest). This may explain some sudden unexpected deaths, including SIDS and unexplained deaths in older children (SUDC), but also some sudden cardiac arrests and those in severe abdominal winding injuries (i.e. kinetic energy diaphragm excitation).
Potent neuromuscular effects of nicotine, a known SIDS risk factor, include excitation and paralysis of the limbs and diaphragm. This shared features with succinylcholine, an anesthetic agent. In nicotine overdoses at all ages, deaths occurred rapidly from respiratory paralysis (peripheral failure, not central). In both, early twitches (fasciculations and spasms) may be followed by muscle contractures. This significantly overlaps with the proposed pathogenesis of DCC and might have been the operative terminal mechanism in SIDS cases where infants were exposed to tobacco smoke. For these reasons, nicotine ingestions and secondhand tobacco smoke exposure are exceptionally dangerous in children.
The causes of diaphragm fatigue are numerous, diverse and complex, and it appears they cumulatively lower the threshold for excitation. Infants are most vulnerable to diaphragm fatigue (and failure) because of higher ventilatory workloads, especially in early infancy (a SIDS risk factor), as well as underdeveloped inspiratory muscles that are comparatively weaker than in their older counterparts (particularly in males, another SIDS risk factor). We speculated SUDC was less common than SIDS because maturation of respiratory muscles protects from critical diaphragm fatigue and failure.
Diaphragm excitation was also proposed to cause some epilepsy deaths (SUDEP), whereby seizure activity transmitted by the phrenic nerves induces diaphragm hyperstimulation and work overload, culminating in fatal DCC apneas. This was supported by shared autopsy findings in SIDS, SUDC and SUDEP as well as continuous diaphragm contraction apneas in epileptic mice [44].
Some obstructive sleep apneas may have a diaphragmatic origin. Respiratory instability in preterm infants (i.e. forced expirations, apneas-hypopneas, hypoxemic episodes, periodic breathing and probably hiccups) and OSA in older children and adults may be caused by spontaneous diaphragm spasms in addition to CNS dysfunction and abdominal muscle contractions (the latter in infants). Yet, many central apnea diagnoses appeared to be erroneous based on diaphragm EMG. Thus, peripheral apneas need to be taken into consideration as a cause of hypoxemic episodes in sleep-disordered breathing at all ages. The best support in infant apneas came from surface EMG demonstrating diaphragm fatigue and temporary failure (Lopes et al. 1981 [4]), as well as intermittent bursts of abdominal electrical activity thought to originate from contractions of the abdominal wall muscles and diaphragm [18,19]. Similarly, in adult sleep apneas, esophageal EMG demonstrated transient electromechanical failure of the diaphragm.
Compelling evidence at SIDS autopsy: Diaphragm histology revealed an anoxic “hypercontraction injury” characteristic of DCC (contraction band necrosis) as well as myofiber disruptions consistent with viral myositis/myopathy, hyperthermia and inspiratory muscle overloading. Along with critical acidosis and hyperkalemia, recently re-reported in SIDS [15], all require further investigation. Autopsy protocols in SIDS and other sudden unexpected deaths should include diaphragm histology, pH, lactate and electrolyte levels.
Increased diaphragmatic muscle tone in acute anxiety might link physical and psychological states at the diaphragm. Anxiety-induced diaphragm hypertonicity and potential squeezing of the esophagus and upper stomach might explain many stress-related gastrointestinal symptoms like acid reflux, vomiting, belching, retching, hiccups and epigastric pain. This was supported by increased hypoxemic-cyanotic events in young children when household stress was present. In addition, “stomach butterflies” might be caused by diaphragm excitation.
Incredulously, thumb-sucking may have spared Patient 0’s infant life by preventing the onset of DCC (which started only after he ceased the habit at age 7). This suggests digit-sucking and pacifiers might reduce diaphragmatic workload (or prevent ventilatory pausing at end-expiration), aligning with their known anxiolytic and SIDS-preventive effects.
Although the evidence presented here is compelling, it is still indirect. Reproducing DCC in laboratory and validating its existence in vivo are urgently needed.
APPENDIX
Supplemental Table S1
Differential diagnosis of Patient 0’s symptoms. Causes of pediatric rib pain (A) and apnea (B) are listed separately and combined (C). Conditions for inclusion in (C), as suggested by the patient's history, were recurrent nocturnal spontaneous sudden onset cramp-like bilateral rib (bearhug) pain with inspiratory arrest. Clinical reasoning yielded six final diagnoses of varying clinical confidence.
Patient’s Perspective and Brief Discussion
This account is written by Patient 0, a practicing medical doctor born in 1970 (53 years old). With trauma counselling, he recently explored painful breathing emergencies that had awakened him from sleep periodically throughout his childhood and youth, always at night while alone in bed. He feels he came within a breath of losing his life each time. He did not tell anyone until now, 45 years later.
“One night when 7 or 8 years old while sleeping in bed alone, my mouth woke me up.
It opened on its own accord, involuntarily.
And when I tried closing it, it did it again, making me wonder just who or what was in command of my now estranged body. Such an odd thing!
Then I bolted upright, realizing I was gasping for air like a fish out of water.
I couldn’t breathe in… at all.
There was an intense pain in my ribs that felt like a tight bearhug.
Still half-asleep, I was convinced my big brother had picked me up from behind, under my arms.
The pain felt like a muscle cramp and was distributed in a C-shape (sternal sparing).
As I fully awoke turning around to yell at him to put me back down, I was shocked to realize I was in my bed. Nobody was there.
All of this took only two or three seconds.
The inability to breathe continued.
I was more stunned and curious than fearful or panicked. However, with the growing sense of doom, I knew I had to act fast. So, I started troubleshooting.
When I tried inhaling more forcefully though, it was met with equal and opposite, complete resistance to airflow. Totally futile, I had to do something different.
Then I tested if I could still exhale.
Yay, something worked!
I remember telling myself not to lose all the air in my lungs just in case I needed to exhale again.
But then came disappointment: I still couldn’t breathe in.
Death approaching, try something else!
This time I exhaled and quickly followed it by three short-burst inhalations with pursed lips (and tongue pressed on hard palate) to increase air pressure [see YouTube Video].
Boom!
The pain stopped and my breathing returned to normal, just like that.
No more air resistance. Completely gone. Crisis averted.
What happened? I almost died! What was that?
And as only a seven-year-old can do, despite a near-death experience, I went back to sleep. [!]
The following morning I was puzzled because the pain had all but disappeared (a hint of sharp pain maybe, but only with deep breathing). I had already learned from an ankle sprain that severe pain like that lasted days, if not longer, but this was gone.
At some point after that, I remember seeing a pilot on TV spinning in a centrifuge using the same distinctive pursed-lip breathing. I had never seen that before. He’s using my trick, I mused.
This was not the end of the breathing emergencies. They sporadically occurred up into my late teens if not later, always at night while fast asleep. I don’t recall how often they happened or if I had any associated illnesses like a cold or flu, however, I did have some diarrhea from time to time over my childhood. The cause was never diagnosed.
With repeated episodes over the years, I eventually recognized in my sleep, prodromal flickering rib pain [fasciculations] to be a warning sign of the impending bearhug apnea like the first episode. This prompted me to quickly take in a breath to prevent it. If I didn’t, the bearhug would come on just at the very end of expiration (just before the next inhalation). I can still recall how the pain always spread from a spot in my right posterolateral ribs to the encircling bearhug in a matter of milliseconds.
The rescue breaths were fairly loud and high pitched, and I remember one night being awakened by the sound… again, my body woke me up! It appears I had grown so accustomed, I did them in my sleep. I also recall telling myself, oddly, to keep it quieter the next time because I didn’t want to wake anybody else up. My memory of that bedroom places it in our family’s newer home, therefore, between ages 17 and 23. I’m not certain if I had any episodes after that.
There are a few childhood medical conditions to share.
Due to severe gastroesophageal reflux, malnourishment and failure to thrive over my first year of life, I underwent an uncomplicated open exploratory laparotomy with Nissen fundoplication at 18 months of age. A congenital hiatal hernia was diagnosed. I recovered well, quickly gained weight and do not recall having reflux after that.
From age 8-9 years, I frequently experience painful fasciculations and muscle cramps that cause contracture-like stiffness of the affected limb. One day, the small muscles of the hand are affected (claw hand), whereas the next involves larger ones like a calf or thigh muscle. With repeated episodes over the years, fasciculations alerted me to prevent imminent cramp by quickly stretching the muscle. I have not received a diagnosis for this ongoing condition.
In addition, starting at 10 years of age, I occasionally become suddenly and extremely fatigued during prolonged or intense exercise. It occurs when not eating properly beforehand. Carbohydrate-rich foods prevent and abort symptoms. This condition was undiagnosed at the time of writing but is consistent with hypoglycemia from McArdle’s (glycogen storage) disease.
Social history: I was the second male, full-term child of a Gravida 4, Para 2 cigarette smoker. Brother denied sleep-disordered breathing, reflux and spontaneous bearhug apneas. I slept alone in an upstairs bedroom of a household containing cigarette smoke that was heated in wintertime.
Notably, I had stopped thumb-sucking around the same time as the onset of the breathing emergencies [important because pacifiers (dummies) are SIDS preventative]. I cannot think of anything else that changed which might explain why it all started at age 7 and not any sooner [during infancy].
In terms of childhood risk factors overlapping with SIDS, mine were numerous including male sex, reflux, chronic diarrhea, residing in a colder climate, household cigarette smoke from maternal use, nocturnal sweating, deep sleeping with preference for the prone position and a tendency to pull bed linens over my shoulders and head sometimes.
I do not have a history of panic attacks, anxiety, depression or sleep disorders such as obstructive sleep apnea, night terrors or sleep paralysis. No cardiac abnormalities such as palpitations, exercise intolerance or syncope. No respiratory issues such as bronchospasm, pneumonia, choking episodes or prolonged cough or colds. No allergies, anaphylaxis or unusual childhood infections. No seizures, tremors, atypical headaches or focal muscle weakness. No family history of cardiac arrhythmias or sudden unexpected deaths, including SIDS.
I survived these life-threatening events because, as opposed to an infant, I had the benefit of wherewithal and ability to problem solve. It is only now, upon reflection as an adult, do I realize how lucky I am to be alive.
I am determined to eradicate DCC. Sleeping children need our help!”
Brief Discussion (re: Patient’s Perspective):
Given such a highly detailed account, it is clear the adult physician/patient was intimately aware of even the finest details surrounding his childhood bearhug pain apneas. The involuntary mouth opening was probably from the gasping reflex, known to occur with severe hypoxemia. From the cramp-like nature and rapid onset and offset of the pain (with near-complete resolution the following morning), it is straightforward to infer spontaneous muscle cramps were causal. Anatomical location suggested it was either the bilateral intercostal muscles or respiratory diaphragm (or both), however, since complete apnea occurred (no inspiratory force), both must have failed. Therefore, simultaneous cramps of the bilateral inspiratory intercostal muscles and diaphragm were proposed. Furthermore, because the cramp induced organ failure, ‘diaphragm cramp-contracture’ was applied (DCC). Upon a literature review, it appeared this abnormality was completely unknown to medicine, and therefore, could be considered novel (see Table: Differential Diagnosis).
The episodic diarrhea may have caused a metabolic acidosis from bicarbonate loss. This acid-base disorder is well known to precipitate and exacerbate limb muscle cramps, particularly during exercise and if dehydrated and overheated. Severe metabolic acidosis also lowers the threshold for ventricular fibrillation, a malignant heart arrhythmia causing sudden cardiac deaths. By extension, acidosis could have contributed to diaphragm excitation in Patient 0. This may have been compounded by his history of generalized muscle cramps (possibly Cramp-Fasciculation Syndrome or some other myopathy).
By initiating expiration to overcome DCC (breathing out to breathe in), this restored respiratory function by forcing the diaphragm out of its hypercontracted state, possibly augmented by the high pressure inspirations. This lifesaving diaphragm movement might have occurred because of the “1-2 punch”-like breathing maneuver: rapid expiration-inspiration could have primed or recoiled the organ in the opposite direction at first, giving a mechanical advantage and moving it more forcefully out of hypercontraction.
As for why the bearhug apneas started at age 7 and not any sooner (during his infancy), something very interesting arises: cessation of his lifelong history of nocturnal thumb-sucking. Indeed, pacifiers (dummies) were found on literature review to be SIDS-preventative [[1]]. Had thumb-sucking protected his life by preventing DCC until age 7? We could only speculate it might have averted respiratory pausing at end-expiration, the point of the respiratory cycle in which he had related the bearhugs were always triggered. Or perhaps it simply reduced diaphragm workload (or both). Unfortunately, no respiratory studies were available, to our knowledge, comparing waveforms in infants sleeping with and without pacifiers.
Indeed, our patient, Patient 0, is very lucky to be alive. Not because of mere chance though. Rather, he clearly had a unique skill set, including breath-holding and logical reasoning, that ultimately enabled grace under intense pressure. We wondered if an adult, under identical circumstances, could do the same thing without panicking.
[1] Li DK, Willinger M, Petitti DB, Odouli R, Liu L, Hoffman HJ. Use of a dummy (pacifier) during sleep and risk of sudden infant death syndrome (SIDS): population based case-control study. BMJ. 2006 Jan 7;332(7532):18-22. PMID: 16339767.
Supplemental Table S2
Why Diaphragm Cramp is Unknown to Medicine. Speculation provided as to how DCC has evaded detection historically.
For a PDF copy of this document, click here to go to Gebien’s website. Comments and feedback welcome.
References
Gebien DJ, Eisenhut M. Uncovering diaphragm cramp in SIDS and other sudden unexpected deaths. Diagnostics (Basel). 2024 Oct 18;14(20):2324. PMID: 39451647.
Goldwater PN. The science (or nonscience) of research into sudden infant death syndrome (SIDS). Front Pediatr. 2022 Apr 15;10:865051. PMID: 35498810.
Siren PM, Siren MJ. Critical diaphragm failure in sudden infant death syndrome. Ups J Med Sci. 2011 May;116(2):115-23. PMID: 21222555.
Lopes JM, Muller NL, Bryan MH, Bryan AC. Synergistic behavior of inspiratory muscles after diaphragmatic fatigue in the newborn. J Appl Physiol Respir Environ Exerc Physiol. 1981 Sep;51(3):547-51. PMID: 7327953.
Dassios T, Vervenioti A, Dimitriou G. Respiratory muscle function in the newborn: a narrative review. Pediatr Res. 2022 Mar;91(4):795-803. PMID: 33875805.
Poets CF, Meny RG, Chobanian MR, Bonofiglo RE. Gasping and other cardiorespiratory patterns during sudden infant deaths. Pediatr Res. 1999Mar;45(3):350-4. PMID: 10088653.
Steinschneider A, Weinstein SL, Diamond E. The sudden infant death syndrome and apnea/obstruction during neonatal sleep and feeding. Pediatrics. 1982 Dec;70(6):858-63. PMID: 7145538.
Kahn A, Groswasser J, Rebuffat E, Sottiaux M, Blum D, Foerster M, et al. Sleep and cardiorespiratory characteristics of infant victims of sudden death: a prospective case-control study. Sleep. 1992 Aug;15(4):287-92. PMID: 1519001.
Jones JM. Solar plexus injuries; an old injury with a new twist. AAO Journal. Ostmed.DR website. 1998. Accessed online 3 December 2024 at https://ostemed-dr.contentdm.oclc.org/digital/collection/myfirst/id/10312/.
Larner AJ. Antony van Leeuwenhoek and the description of diaphragmatic flutter (respiratory myoclonus). Mov Disord. 2005 Aug;20(8):917-8. PMID: 16007667.
Adams JA, Zabaleta IA, Sackner MA. Diaphragmatic flutter in three babies with bronchopulmonary dysplasia and respiratory syncytial virus bronchiolitis. Pediatr Pulmonol. 1995 May;19(5):312-6. PMID: 7567207.
Katz ES, Gauda E, Crawford T, Ogunlesi F, Lefton-Greif MA, McGrath-Morrow S, Marcus CL. Respiratory flutter syndrome: an underrecognized cause of respiratory failure in neonates. Am J Respir Crit Care Med. 2001 Oct 1;164(7):1161-4. PMID: 11673203.
Shi Z, Bogaards SJP, Conijn S, Onderwater Y, Espinosa P, Bink DI, et al. COVID-19 is associated with distinct myopathic features in the diaphragm of critically ill patients. BMJ Open Respir Res. 2021 Sep;8(1):e001052. PMID: 34544735.
Davis GG, Glass JM. Case report of sudden death after a blow to the back of the neck. Am J Forensic Med Pathol. 2001 Mar;22(1):13-8. PMID: 11444655.
Goldwater PN, Gebien DJ. Metabolic acidosis and sudden infant death syndrome: overlooked data provides insight into SIDS pathogenesis.World J Pediatr. 2024 Dec 10. PMID: 39656413.
Gebien DJ. Myositis “diaphragm cramp” as a potential cause of respiratory arrests in infants. Comment on Salfi NCM. et al. Fatal deterioration of a respiratory syncytial virus infection in an infant with abnormal muscularization of intra-acinar pulmonary arteries: autopsy and histological findings. Diagnostics 2024, 14, 601. Diagnostics. 2024; 14(10):1061. PMID: 38786359.
Southall DP, Samuels MP, Talbert DG. Recurrent cyanotic episodes with severe arterial hypoxaemia and intrapulmonary shunting: a mechanism for sudden death. Arch Dis Child. 1990 Sep;65(9):953-61. PMID: 2221968.
Esquer C, Claure N, D'Ugard C, Wada Y, Bancalari E. Role of abdominal muscles activity on duration and severity of hypoxemia episodes in mechanically ventilated preterm infants. Neonatology. 2007;92(3):182-6. PMID: 17476118.
Abu-Osba YK, Brouillette RT, Wilson SL, Thach BT. Breathing pattern and transcutaneous oxygen tension during motor activity in preterm infants. Am Rev Respir Dis. 1982 Apr;125(4):382-7. PMID: 7073106.
Brouillette, R.T.; Thach, B.T.; Abu-Osba, Y.K.; Wilson, S.L. Hiccups in infants: characteristics and effects on ventilation. J. Pediatr. 1980, 96, 219–225.PMID: 7351583.
Zhang S, Li B, Zhang X, Zhu C, Wang X. Birth asphyxia is associated with increased risk of cerebral palsy: a meta-analysis. Front Neurol. 2020 Jul 16;11:704. PMID: 32765409.
LaRosa DA, Ellery SJ, Parkington HC, Snow RJ, Walker DW, Dickinson H. Maternal creatine supplementation during pregnancy prevents long-term changes in diaphragm muscle structure and function after birth asphyxia. PLoS One. 2016 Mar 1;11(3):e0149840. PMID: 26930669.
Poets CF, Roberts RS, Schmidt B, Whyte RK, Asztalos EV, Bader D, et al; Canadian Oxygen Trial Investigators. Association between intermittent hypoxemia or bradycardia and late death or disability in extremely preterm infants. JAMA. 2015 Aug 11;314(6):595-603. PMID: 26262797.
Miguel PM, Schuch CP, Rojas JJ, Carletti JV, Deckmann I, Martinato LH, et al. Neonatal hypoxia-ischemia induces attention-deficit hyperactivity disorder-like behavior in rats. Behav Neurosci. 2015 Jun;129(3):309-20. PMID: 26030430.
Miller MJ, Petrie TG, Difiore JM. Changes in resistance and ventilatory timing that accompany apnea in premature infants. J Appl Physiol (1985). 1993 Aug;75(2):720-3. PMID: 8226474.
Steier J, Jolley CJ, Seymour J, Ward K, Luo YM, Polkey MI, Moxham J. Increased load on the respiratory muscles in obstructive sleep apnea. Respir Physiol Neurobiol. 2010 Apr 15;171(1):54-60. PMID: 20117253.
Luo YM, Tang J, Jolley C, Steier J, Zhong NS, Moxham J, Polkey MI. Distinguishing obstructive from central sleep apnea events: diaphragm electromyogram and esophageal pressure compared. Chest. 2009 May;135(5):1133-1141. PMID: 19118271.
Townsel CD, Emmer SF, Campbell WA, Hussain N. Gender differences in respiratory morbidity and mortality of preterm neonates. Front Pediatr. 2017Jan 30;5:6. PMID: 28194395.
Surén P, Bakken IJ, Aase H, Chin R, Gunnes N, Lie KK, et al. Autism spectrum disorder, ADHD, epilepsy, and cerebral palsy in Norwegian children. Pediatrics. 2012 Jul;130(1):e152-8. PMID: 22711729.
Yeung T, Ibrahim J, Mohamed A. Sex-based differences in the sonographic characterization of diaphragm thickness in preterm infants with bronchopulmonary dysplasia at term corrected age: a secondary analysis of a prospective study. J Ultrasound Med. 2024 Feb 20. PMID: 38375956.
Agyeman P, Duppenthaler A, Heininger U, Aebi C. Influenza-associated myositis in children. Infection. 2004 Aug;32(4):199-203. PMID: 15293074.
Kariks J. Diaphragmatic muscle fibre necrosis in SIDS. Forensic Sci Int. 1989 Dec;43(3):281-91. PMID: 2613142.
Eisenhut M. Features of diaphragmatic myositis in a case of sudden infant death. Ups J Med Sci. 2011 Aug;116(3):220. PMID: 21671726.
Sundararajan S, Ostojic NS, Rushton DI, Cox PM, Acland P. Diaphragmatic pathology: a cause of clinically unexplained death in the perinatal/paediatric age group. Med Sci Law. 2005 Apr;45(2):110-4. PMID: 15895635.
Jiang TX, Reid WD, Belcastro A, Road JD. Load dependence of secondary diaphragm inflammation and injury after acute inspiratory loading. Am J Respir Crit Care Med. 1998 Jan;157(1):230-6. PMID: 9445304.
Orozco-Levi M, Lloreta J, Minguella J, Serrano S, Broquetas JM, Gea J. Injury of the human diaphragm associated with exertion and chronic obstructive pulmonary disease. Am J Respir Crit Care Med. 2001 Nov 1;164(9):1734-9. PMID: 11719318.
Goldwater PN. Sudden infant death syndrome: a critical review of approaches to research. Arch Dis Child. 2003 Dec;88(12):1095-100. PMID: 14670779.
Mychaleckyj JC, Normeshie C, Keene KL, Hauck FR. Organ weights and length anthropometry measures at autopsy for sudden infant death syndrome cases and other infant deaths in the Chicago infant mortality study. Am J Hum Biol. 2024 Oct;36(10):e24126. PMID: 38957054.
Fogarasi A, Janszky J, Tuxhorn I. Autonomic symptoms during childhood partial epileptic seizures. Epilepsia. 2006 Mar;47(3):584-8. PMID: 16529626.
Southall DP, Talbert DG, Johnson P, Morley CJ, Salmons S, Miller J, Helms PJ. Prolonged expiratory apnoea: a disorder resulting in episodes of severe arterial hypoxaemia in infants and young children. Lancet. 1985 Sep 14;2(8455):571-7. PMID: 2863595.
Rumajogee P, Bregman T, Miller SP, Yager JY, Fehlings MG. Rodent hypoxia-ischemia models for cerebral palsy research: a systematic review. Front Neurol. 2016 Apr 25;7:57. PMID: 27199883.
Preciado C, Baida M, Li Y, Li Y, Demopoulos C. Prenatal exposure to hypoxic risk conditions in autistic and neurotypical youth: Associated ventricular differences, sleep disturbance, and sensory processing. Autism Res. 2024 Oct 16. PMID: 39411851.
Zhang X, Zhang J, Wang J, Zou D, Li Z. Analysis of forensic autopsy cases associated with epilepsy: Comparison between sudden unexpected death in epilepsy (SUDEP) and not-SUDEP groups. Front Neurol. 2022 Dec 9;13:1077624. PMID: 36570468.
Wenker IC, Teran FA, Wengert ER, et al. Postictal death is associated with tonic phase apnea in a mouse model of sudden unexpected death in epilepsy. Ann Neurol. 2021 May;89(5):1023-1035. PMID: 33604927.